Medical expert of the article
New publications
Preparations
Inhalation anesthetics
Last reviewed: 23.04.2024
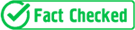
All iLive content is medically reviewed or fact checked to ensure as much factual accuracy as possible.
We have strict sourcing guidelines and only link to reputable media sites, academic research institutions and, whenever possible, medically peer reviewed studies. Note that the numbers in parentheses ([1], [2], etc.) are clickable links to these studies.
If you feel that any of our content is inaccurate, out-of-date, or otherwise questionable, please select it and press Ctrl + Enter.
General anesthesia is defined as drug-induced reversible CNS depression, leading to a lack of response of the body to external stimuli.
The history of the use of inhalational anesthetics as a means of general anesthesia began with a public demonstration in 1846 of the first etheric anesthesia. In the 1940s, dinitrogen oxide (Wells, 1844) and chloroform (Simpson, 1847) were introduced into practice. These inhalation anesthetics were used until the mid-50s of the 20th century.
In 1951, halothane was synthesized, which began to be used in the anesthetic practice of many countries, incl. And in the domestic. Approximately in the same years, methoxyflurane was obtained, but because of too high solubility in blood and tissues, slow induction, prolonged elimination and nephrotoxicity, the drug is now of historical significance. Hepatotoxicity of halothane led to the search for new halogen-containing anesthetics, which in the 70s led to the creation of three drugs: enflurane, isoflurane and sevoflurane. The latter, despite its high cost, was spread due to low solubility in tissues and pleasant odor, good tolerability and rapid induction. Finally, the last drug of this group, desflurane, was introduced into clinical practice in 1993. Desflurane has an even lower solubility in tissues than sevoflurane, and thus provides excellent control over the maintenance of anesthesia. When compared with other anesthetics in this group, desflurane has the fastest way out of anesthesia.
Quite recently, already at the end of the 20th century, an anesthetic practice included a new gaseous anesthetic - xenon. This inert gas is a natural component of the heavy air fraction (for every 1000 m3 of air there are 86 cm3 of xenon). The use of xenon in medicine until recently was limited to the field of clinical physiology. Radioactive isotopes 127Xe and 111Xe were used to diagnose diseases of the respiratory organs, blood circulation, and organ blood flow. The narcotic properties of xenon were predicted (1941) and confirmed (1946) by N.V. Lazarev. The first use of xenon in the clinic dates back to 1951 (S. Cullen and E. Gross). In Russia, the use of xenon and its further study as a means for anesthesia is associated with the names of L.A. Buachidze, V.P. Smolnikova (1962), and later N.E. Burov. The monograph by N.E. Burov (together with VN Potapov and GA Makeev) "Xenon in anesthesiology" (clinical and experimental research), published in 2000, is the first in world anesthesia practice.
Currently, inhalational anesthetics are used mainly during the period of maintenance of anesthesia. For the purpose of introductory anesthesia, inhalational anesthetics are used only in children. Today in the arsenal of the anesthetist there are two gaseous inhalation anesthetics - dinitrogen oxide and xenon and five liquid substances - halothane, isoflurane, enflurane, sevoflurane and desflurane. Cyclopropane, trichlorethylene, methoxyflurane and ether are not used in the clinical practice of most countries. Diethyl ether is still used in selected small hospitals in the Russian Federation. The specific weight of various methods of general anesthesia in modern anesthesia is up to 75% of the total number of anesthesia, the remaining 25% fall on different variants of local anesthesia. Inhalation methods of general anesthesia dominate. In / in methods of general anesthesia are approximately 20-25%.
Inhaled anesthetics in modern anesthesiology are used not only as drugs for mononarcosis, but also as components of a general balanced anesthesia. The very idea - to use small doses of drugs that will potentiate each other and give an optimal clinical effect, was quite revolutionary in the era of mononarcosis. In fact, it was at this time that the principle of multi-component modern anesthesia was implemented. Balanced anesthesia solved the main problem of that period - an overdose of a narcotic substance due to the lack of precise evaporators.
Dinitrogen oxide was used as the main anesthetic, barbiturates and scopolamine provided sedation, belladonna and opiates inhibited reflex activity, opioids caused analgesia.
Today, for balanced anesthesia, along with dinitrogen oxide, xenon or other modern inhalation anesthetics are used, benzodiazepines replaced barbiturates and scopolamine, old analgesics gave way to modern (fentanyl, sufentanil, remifentanil), and new muscle relaxants appeared that minimally affect vital organs. Neuro-vegetative braking was begun with neuroleptics and clonidine.
Inhalation anesthetics: a place in therapy
The era of mononarcosis disappears with the help of this or that inhalational anesthetic. Although in pediatric practice and with small-scale surgical operations in adults, this technique is still practiced. Multicomponent general anesthesia dominates anesthesia practice since the 60s of the last century. The role of inhalational anesthetics is limited to the achievement and maintenance of the first component - the switching off of consciousness and maintenance of the narcotic state during surgical intervention. The depth of anesthesia should correspond to 1.3 MAC of the selected drug, taking into account all the additional adjuvants used that affect the MAC. An anesthesiologist should keep in mind that the inhalation component has a dose-dependent effect on other components of general anesthesia, such as analgesia, muscle relaxation, neurovegetative inhibition, etc.
Introduction to anesthesia
The question of introduction into anesthesia today can be said to have been resolved in favor of intravenous anesthetics with the subsequent transition to an inhalation component in order to maintain anesthesia. At the heart of this decision, of course, is comfort for the patient and the speed of induction. However, it should be borne in mind that in the transitional stage from the initial anesthesia to the maintenance period, there are several pitfalls associated with inadequate anesthesia and, as a result, the reaction of the body to the endotracheal tube or skin incision. This is often observed when an anesthesiologist uses for the induction anesthesia barbiturates of ultrashort action or hypnotics, devoid of analgesic properties, and does not have time to saturate the body with an inhalational anesthetic or a strong analgesic (fentanyl). Hyperdynamic blood circulation reaction accompanying this condition can be extremely dangerous in elderly patients. Preliminary introduction of muscle relaxants makes a violent response of the patient invisible. However, the indicators monitor the "vegetative storm" from the cardiovascular system. It is during this period that awakening of patients with all the negative consequences of this condition is often observed, especially if the operation has already begun.
There are several options to prevent the inclusion of consciousness and smooth achievement of the maintenance period. This is a timely saturation of the body with inhalational anesthetics, which allow to reach the MAC or better than UHF5 by the end of the IV action of the injecting agent. Another option may be a combination of inhalation anesthetics (dinitrogen oxide + isoflurane, sevoflurane or xenon).
A good effect is observed when benzodiazepines are combined with ketamine, dinitrogen oxide with ketamine. Confidence in the anesthetist is given by the additional administration of fentanyl and muscle relaxants. Combined methods are common, when inhalation agents are combined with IV. Finally, the use of strong inhalation anesthetics of sevoflurane and desflurane, which have a low solubility in blood, allows rapid drug concentrations to be reached before the introductory anesthetic ceases to function.
Mechanism of action and pharmacological effects
Despite the fact that about 150 years have passed since the first etheric anesthesia was given, the mechanisms of the narcotic effect of inhalational anesthetics are not completely clear. The existing theories (coagulation, lipoid, surface tension, adsorption), proposed in the late XIX and early XX centuries, could not reveal the complex mechanism of general anesthesia. Likewise, the theory of water microcrystals of the twice Nobel Laureate L. Pauling did not answer all questions. In the opinion of the latter, the development of the narcotic state is explained by the property of general anesthetics to form in the aqueous phase of tissues original crystals that create an obstacle for the movement of cations through the cell membrane and thereby block the process of depolarization and the formation of the action potential. In subsequent years, studies appeared that showed that not all anesthetics have the property of forming crystals, and those that have this property form crystals at concentrations that exceed the clinical values. In 1906, the English physiologist C. Sherrington suggested that general anesthetics realize their specific action mainly through synapses, exerting a retarding effect on the synaptic transmission of excitation. However, the mechanism of inhibition of neuronal excitability and inhibition of synaptic transmission of excitation under the influence of anesthetics is not fully disclosed. According to some scientists, the molecules of the anesthetic form on the membrane of the neuron a kind of cloak that hampers the passage of ions through it and thereby prevents the membrane depolarization process. According to other researchers, anesthetics alter the function of the cation "channels" of cell membranes. It is obvious that different anesthetics unequally affect the main functional links of the synapses. Some of them inhibit the transmission of excitation primarily at the level of the terminals of nerve fibers, others - reduce the sensitivity of membrane receptors to the mediator or inhibit its formation. The antinociceptive system of the body can serve as a confirmation of the primary effect of general anesthetics in the zone of inter-neuron contacts, which in modern terms is a set of mechanisms regulating pain sensitivity and exerting a retarding effect on nociceptive impulses in general.
The concept of a change in the physiological lability of neurons and especially synapses under the influence of narcotic substances has made it possible to come to an understanding that at any given moment of general anesthesia the degree of inhibition of the function of different parts of the brain is not the same. This understanding was confirmed by the fact that along with the cortex of the cerebral hemispheres, the function of the reticular formation, which was the most susceptible to the inhibitory effect of narcotic substances, was a prerequisite for the development of the "reticular theory of anesthesia." Confirmation of this theory was the evidence that the destruction of certain zones of the reticular formation caused a state close to drug-induced sleep or anesthesia. To date, the idea has been formed that the effect of general anesthetics is the result of inhibition of reflex processes at the level of the reticular substance of the brain. This eliminates its upward activating influence, which leads to deafferentation of the overlying sections of the central nervous system. With all the popularity of the "reticular theory of anesthesia," it can not be recognized as universal.
Admittedly, much has been done in this area. However, there are still questions for which there are no reliable answers.
Minimal alveolar concentration
The term "minimal alveolar concentration" (MAK) was introduced in 1965 by Eger et al. As a standard of potency (strength, power) of anesthetics. This MAK inhalation anesthetics, preventing motor activity in 50% of subjects who are given a pain stimulus. MAC for each anesthetic is not a static value and can vary depending on the patient's age, ambient temperature, interaction with other medicines, the presence of alcohol, etc.
For example, the introduction of narcotic analgesics and sedative drugs reduces the MAC. Conceptually, between MAK and the average effective dose (ED50), a parallel can be drawn in exactly the same way as ED95 (no movement to a pain stimulus in 95% of patients) is equivalent to 1.3 MAK.
Minimal alveolar concentration of inhalational anesthetics
- Dinitrogen oxide - 105
- Xenon - 71
- Hapotan - 0.75
- Enflurane - 1.7
- Isoflurane - 1,2
- Sevoflurane - 2
- Desflurane - 6
To achieve the MAC = 1, hyperbaric conditions are necessary.
The addition of 70% of the dinitrogen oxide, or nitrous oxide (N20), to enflurane reduces the MAC of the latter from 1.7 to 0.6, to halotane from 0.77 to 0.29, to isoflurane from 1.15 to 0.50 , to sevoflurane - from 1.71 to 0.66, to desflurane - from 6.0 to 2.83. Decreased MAK, in addition to the causes mentioned above, metabolic acidosis, hypoxia, hypotension, a2-agonists, hypothermia, hyponatremia, hypoosmolarity, pregnancy, alcohol, ketamine, opioids, muscle relaxants, barbiturates, benzodiazepines, anemia, etc.
The following factors do not influence the MAC: the duration of anesthesia, hypo- and hypercarbia within PaC02 = 21-95 mm Hg. Metabolic alkalosis, hyperoxia, arterial hypertension, hyperkalemia, hyperosmolarity, propranolol, isoproterenol, naloxone, aminophylline, etc.
Influence on the central nervous system
Inhalational anesthetics cause very significant changes at the level of the central nervous system: the deactivation of consciousness, electrophysiological disorders, changes in cerebral hemodynamics (cerebral blood flow, brain oxygen consumption, pressure of cerebrospinal fluid, etc.).
When inhaled inhalation anesthetics with increasing doses violated the relationship between cerebral blood flow and brain oxygen consumption. It is important to keep in mind that this effect is observed when cerebral vascular autoregulation is intact against a background of normal intracranial arterial pressure (BP) (50-150 mm Hg). An increase in cerebral vasodilation with a subsequent increase in cerebral blood flow leads to a decrease in brain oxygen consumption. This effect decreases or disappears with a decrease in blood pressure.
Each strong inhalation anesthetic reduces the metabolism of brain tissue, causes vasodilation of cerebral vessels, increases the pressure of cerebrospinal fluid and the cerebral volume of blood. Dinitrogen oxide moderately increases the total and regional cerebral blood flow, so there is no significant increase in intracranial pressure. Xenon also does not increase intracranial pressure, but compared with 70% dinitrogen oxide, it almost doubles the rate of cerebral blood flow. Restoration of the old parameters occurs immediately after the gas supply has ceased.
In the waking state, cerebral blood flow is clearly correlated with brain oxygen consumption. If the intake decreases, the cerebral blood flow also decreases. Isoflurane can maintain this correlation dependence better than other anesthetics. An increase in cerebral blood flow with anesthetics tends to gradually normalize to the initial level. In particular, after an initial anesthesia with halothane, the cerebral blood flow is normalized within 2 hours.
Inhalational anesthetics have a significant effect on the volume of cerebrospinal fluid, affecting both its production and its reabsorption. So, if enflurane increases the production of cerebrospinal fluid, then isoflurane does not affect almost neither the product nor the reabsorption. Halothane also reduces the production rate of cerebrospinal fluid, but increases the resistance to reabsorption. In the presence of moderate hypocapnia, it is less likely that isoflurane will cause a dangerous increase in cerebrospinal pressure compared to halothane and enflurane.
Inhalational anesthetics have a significant effect on the electroencephalogram (EEG). With an increase in the concentration of anesthetics, the frequency of bioelectric waves decreases and their voltage increases. At very high concentrations of anesthetics, there may be zones of electrical silence. Xenon, like other anesthetics, at a concentration of 70-75% causes depression of alpha and beta activity, reduces the frequency of the EEG oscillations to 8-10 Hz. Inhaling 33% of xenon for 5 minutes to diagnose the state of cerebral blood flow causes a number of neurological disorders: euphoria, dizziness, respiratory arrest, nausea, numbness, stupor, heaviness in the head. The decrease in the amplitude of alpha and beta waves noted at this time is of a transient nature, and the EEG is restored after the xenon feed is stopped. According to N.E. Burov et al. (2000), no negative effects of xenon on brain structures and its metabolism were noted. Unlike other inhalation anesthetics, enflurane can cause a high-amplitude repeated acute-pointed wave activity. This activity can be leveled by a decrease in the dose of enflurane or an increase in PaCOa.
Influence on the cardiovascular system
All strong inhalation anesthetics inhibit the cardiovascular system, but their hemodynamic effect is different. The clinical manifestation of cardiovascular depression is hypotension. In particular, in halothane, this effect is mainly due to a decrease in the contractility of the myocardium and the frequency of its contractions with a minimal decrease in total vascular resistance. Enflurane also causes depression of myocardial contractility, and reduces the overall peripheral resistance. In contrast to halothane and enflurane, the effect of isoflurane and desflurane is mainly due to a decrease in vascular resistance and is dose-dependent. With an increase in the concentration of anesthetics up to 2 MAK, blood pressure can be reduced by 50%.
A negative chronotropic effect is characteristic of halothane, whereas enflurane often causes tachycardia.
Data from experimental studies by Skovster et al., 1977, have shown that isoflurane inhibits both vagal and sympathetic functions, however, because the vagal structures are more severely inhibited, there is an increase in the rhythm of the heart. It should be pointed out that a positive chronotropic effect is more often observed in young subjects, and in patients after 40 years its severity decreases.
Cardiac output is reduced mainly by reducing the stroke volume with halothane and enflurane and to a lesser extent isoflurane.
Halothane has the least influence on the rhythm of the heart. Desflurane causes the most pronounced tachycardia. Due to the fact that blood pressure and cardiac output either decrease or remain stable, the work of the heart and oxygen consumption by oxygen by 10-15% decrease.
Dinitrogen oxide affects hemodynamics variable. In patients with heart disease, dinitrogen oxide, especially when combined with opioid analgesics, causes hypotension and a decrease in cardiac output. This does not occur in young subjects with a normally functioning cardiovascular system, where the activation of the sympathoadrenal system neutralizes the depressive effect of the dinitrogen oxide on the myocardium.
The effect of the oxide dinitrogen on a small circle is also variable. In patients with increased pressure in the pulmonary artery, the addition of dinitrogen oxide can further increase it. It is interesting to note that the decrease in pulmonary vascular resistance with isoflurane is less than the decrease in systemic vascular resistance. Sevoflurane affects hemodynamics to a lesser extent than isoflurane and desflurane. According to the literature, xenon favorably affects the cardiovascular system. There is a tendency to bradycardia and a slight increase in blood pressure.
Anesthetics have a direct effect on the hepatic circulation and vascular resistance in the liver. In particular, if isoflurane causes vasodilation of the blood vessels of the liver, halothane does not have this effect. Both reduce total hepatic blood flow, but the need for oxygen is lower with isoflurane anesthesia.
Addition of the dinitrogen oxide to halothane contributes to a further decrease in the celiac blood flow, and isoflurane may interfere with renal and celiac vasoconstriction associated with somatic or visceral neural stimulation.
Influence on the rhythm of the heart
Cardiac arrhythmias can occur in more than 60% of patients under conditions of inhalation anesthesia and surgery. Enflurane, isoflurane, desflurane, sevoflurane, dinitrogen oxide and xenon are less likely to cause rhythm disturbances than halothane. Arrhythmias associated with hyperadrenalinemia, in conditions of halothane anesthesia, are more pronounced in adults than in children. Arrhythmias are promoted by hypercarbia.
Atrioventricular nodal rhythm is often observed with inhalation of almost all anesthetics, perhaps, with the exception of xenon. This is especially pronounced in anesthesia with enflurane and dinitrogen oxide.
Coronary autoregulation provides an equilibrium between the coronary blood flow and the need for myocardium in oxygen. In patients with ischemic heart disease (IHD) under conditions of isoflurane anesthesia, coronary blood flow does not decrease, despite a decrease in systemic blood pressure. If hypotension is caused by isoflurane, then in the presence of experimental stenosis of the coronary artery in dogs, marked myocardial ischemia occurs. If hypotension can be prevented, then isoflurane does not cause stealing syndrome.
At the same time, dinitrogen oxide, added to a strong inhalation anesthetic, can disrupt the distribution of coronary blood flow.
Kidney blood flow in conditions of general inhalation anesthesia does not change. This is facilitated by autoregulation, which reduces the overall peripheral resistance of the renal vessels if the systemic blood pressure decreases. The rate of glomerular filtration decreases because of the decrease in blood pressure, and as a result, the production of urine decreases. When restoring the blood pressure, everything returns to the original level.
Influence on the respiratory system
All inhalation anesthetics have a depressive effect on respiration. With increasing dose, breathing becomes superficial and frequent, the volume of inspiration decreases, and the tension of carbon dioxide in the blood increases. However, not all anesthetics increase the respiratory rate. Thus, isoflurane only in the presence of dinitrogen oxide can lead to increased rates of respiration. Xenon also attenuates breathing. When the concentration reaches 70-80%, respiration is reduced to 12-14 per min. It should be borne in mind that xenon is the heaviest gas of all inhalation anesthetics and has a density factor of 5.86 g / l. In this regard, the addition of narcotic analgesics during xenon anesthesia, when the patient breathes independently, is not shown. According to Tusiewicz et al., 1977, respiratory efficiency is 40% achieved by intercostal muscles and 60% by diaphragm. Inhalational anesthetics have a dose-dependent depressive effect on these muscles, which increases significantly when combined with narcotic analgesics or drugs that have a central muscle relaxant effect. With inhalation anesthesia, especially when the concentration of anesthetic is high enough, apnea may occur. And the difference between MAK and the dose caused by apnea is different for anesthetics. The lowest is for enflurane. Inhalational anesthetics have a unidirectional effect on the tone of the airways - they reduce the resistance of the respiratory tract due to bronchodilation. This effect in halothane is more pronounced than that of isoflurane, enflurane, and sevoflurane. Therefore, it can be concluded that all inhalational anesthetics are effective in patients with bronchial asthma. However, their effect is due not to the blocking of histamine release, but to the prevention of the bronchoconstrictive effect of the latter. It should also be remembered that inhalation anesthetics to some extent inhibit mucociliary activity, which, together with such negative factors as finding the endotracheal tube and inhalation of dry gases, creates the conditions for the occurrence of postoperative bronchopulmonary complications.
Effects on liver function
In connection with the rather high (15-20%) metabolism of halothane in the liver, the opinion about the possibility of a hepatotoxic effect of the latter has always existed. And although in the literature single cases of liver damage were described, this danger took place. Therefore, the synthesis of subsequent inhalation anesthetics had the main goal - to reduce the hepatic metabolism of new halogen-containing inhalation anesthetics and to reduce hepatotoxic and nephrotoxic effects to a minimum. And if methoxyfluorane has a 40-50% metabolism, 15-20% in halothane, 3% in sevoflurane, 2% in enflurane, 0.2% in isoflurane, and 0.02% in desflurane. The data presented indicate that desflurane does not have a hepatotoxic effect, in isoflurane it is only theoretically possible, and in enflurane and sevoflurane it is extremely low. A million sevoflurane anesthetics performed in Japan described only two cases of liver damage.
[7], [8], [9], [10], [11], [12]
Effect on blood
Inhalational anesthetics affect hematopoiesis, cellular elements and coagulation. In particular, the teratogenic and mielodepressive effects of the oxide dinitrogen are well known. The prolonged exposure of the dinitrogen oxide causes anemia due to inhibition of the enzyme methionine synthetase, which is included in the metabolism of vitamin B12. Megaloblastic changes in the bone marrow were detected even after a 105-minute inhalation of the clinical concentration of dinitrogen oxide in severe patients.
There are indications that inhalation anesthetics affect platelets and thus contribute to bleeding, either by affecting the smooth muscle of the vessels, or by affecting the function of platelets. There is evidence that halothane reduces their ability to aggregate. A moderate increase in bleeding was noted during halothane anesthesia. This phenomenon was absent in inhalation of isoflurane and enflurane.
Influence on the neuromuscular system
It has long been known that inhalational anesthetics potentiate the action of muscle relaxants, although the mechanism of this effect is not clear. In particular, it was found that isoflurane potentiates the succinylcholine block more than halothane. At the same time, it was noted that inhalational anesthetics cause a greater degree of potentiation of nondepolarizing muscle relaxants. There is a definite difference between the effects of inhalational anesthetics. For example, isoflurane and enflurane potentiate a neuromuscular blockage of greater length than halothane and sevoflurane.
Influence on the endocrine system
During anesthesia, the glucose level rises either as a result of a decrease in insulin secretion, or because of a decrease in the ability of peripheral tissues to utilize glucose.
Of all the inhaled anesthetics, sevoflurane maintains the glucose concentration at baseline, and therefore sevoflurane is recommended for use in diabetic patients.
The assumption that inhalation anesthetics and opioids caused the secretion of antidiuretic hormone, was not confirmed by more accurate methods of research. It was found that a significant release of antidiuretic hormone is part of the stress response to surgical stimulation. Little is affected by inhalation anesthetics and the level of renin and serotonin. At the same time, it has been established that halothane significantly reduces the level of testosterone in the blood.
It is noted that inhalation anesthetics during induction have a greater effect on the release of hormones (adrenocorticotropic, cortisol, catecholamines) than drugs for intravenous anesthesia.
Halothane more than enflurane, increases the level of catecholamines. In connection with the fact that halothane increases the sensitivity of the heart to adrenaline and promotes arrhythmias, the use of enflurane, isoflurane and sevoflurane is more indicated when the pheochromocytoma is removed.
Effects on the uterus and fetus
Inhalational anesthetics cause miometralnuyu relaxation and thereby increase perinatal blood loss. Compared to anesthesia with dinitrogen oxide in combination with opioids, blood loss after halothane, enflurane and isoflurane anesthesia is significantly higher. However, the use of small doses of 0.5% halothane, 1% enflurane, and 0.75% isoflurane as an adjunct to narcosis with dinitrogen oxide and oxygen, on the one hand, prevents awakening on the operating table, on the other - does not significantly affect blood loss.
Inhalation anesthetics penetrate the placenta and affect the fetus. In particular, 1 MAC galothane causes hypotension in the fetus even with minimal hypotension and tachycardia in the mother. However, this hypotension in the fetus is accompanied by a decrease in peripheral resistance, and as a result, peripheral blood flow remains at a sufficient level. Nevertheless, it is safer for the fetus to use isoflurane.
Pharmacokinetics
The entry of a gaseous or vaporous anesthetic directly into the patient's lungs facilitates the rapid diffusion of drugs from the pulmonary alveoli into the arterial blood and then its distribution to vital organs, creating a certain concentration of drugs in them. The severity of the effect ultimately depends on the achievement of the therapeutic concentration of an inhalational anesthetic in the brain. Since the latter is an extremely well perfused organ, the partial pressure of the inhalation agent in the blood and brain is leveled fairly quickly. The exchange of the inhalation anesthetic through the alveolar membrane is very effective, so the partial pressure of the inhalation agent in the blood circulating through a small circle is very close to what is found in the alveolar gas. Thus, the partial pressure of the inhalation anesthetic in the brain tissues differs little from the alveolar partial pressure of the same agent. The reason why a patient does not fall asleep immediately after the onset of inhalation and does not wake up immediately after its discontinuation is mainly the solubility of the inhalation anesthetic in the blood. The penetration of drugs into their place of action can be represented in the following stages:
- evaporation and entry into airways;
- transition through the alveolar membrane and entry into the blood;
- transition from blood through the tissue membrane into the cells of the brain and other organs and tissues.
The rate of ingestion of an inhaled anesthetic from the alveoli to the blood depends not only on the solubility of the anesthetic in the blood, but also on the alveolar blood flow and the difference in the partial pressures of the alveolar gas and venous blood. Before reaching narcotic concentration, the inhalation agent passes the path: alveolar gas -> blood -> brain -> muscles -> fat, i.e. From well-vascularized organs and tissues to poorly vascularized tissues.
The higher the blood / gas ratio, the higher the solubility of the inhalation anesthetic (Table 2.2). In particular, it is obvious that if halothane has a blood / gas growth rate of 2.54, and desflurane is 0.42, desflurane's initial anesthesia is 6 times faster than halothane. If you compare the latter with methoxyflurane, whose blood / gas ratio is 12, then it becomes clear why methoxyfluorane is not suitable for induction anesthesia.
The amount of anesthetic that undergoes liver metabolism is significantly less than exhaled through the lungs. The percentage of methoxyfluor metabolism is 40-50%, halothane 15-20%, sevoflurane 3%, en fluran 2%, isoflurane 0.2%, and desflurane 0.02%. Diffusion of anesthetics through the skin is minimal.
When the supply of anesthetic ceases, its elimination begins on the principle opposite to induction. The lower the solubility factor of the anesthetic in the blood and tissues, the faster the awakening. Quick elimination of the anesthetic is facilitated by a high oxygen flow and, accordingly, high alveolar ventilation. Elimination of the dinitrogen of oxide and xenon passes so quickly that diffusion hypoxia can occur. The latter can be prevented by inhalation of 100% oxygen for 8-10 minutes under the control of the percentage of anesthetic in the blown air. Of course, that the speed of awakening depends on the duration of use of the anesthetic.
Lead-out period
The exit from anesthesia in modern anesthesiology is predictable enough if the anesthesiologist has sufficient knowledge in the field of clinical pharmacology of the drugs used. The rate of awakening depends on a number of factors: the dose of drugs, its pharmacokinetics, the patient's age, the duration of anesthesia, blood loss, the number of transfused oncotic and osmotic solutions, the patient's temperature and the environment, etc. In particular, the difference in the speed of awakening with desflurane and sevoflurane is 2 times faster than with isoflurane and halothane. The latter drugs also have an advantage over ether and methoxy flurane. And yet, the most controlled inhalation anesthetics last longer than some intravenous anesthetics, for example propofol, and patients awaken within 10-20 minutes after discontinuation of the inhalation anesthetic. Of course, the calculation should take all drugs that were introduced during anesthesia.
Maintaining anesthesia
Anesthesia can be maintained with the help of an inhalation anesthetic alone. However, many anesthetists still prefer to add adjuvant to the background of the inhalation agent, in particular analgesics, relaxants, antihypertensives, cardiotonics, etc. Having in its arsenal inhalation anesthetics with different properties, the anesthetist can choose an agent with the necessary properties and use not only its narcotic properties, but also, for example, the hypotensive or bronchodilating effect of anesthetic. In neurosurgery, for example, prefer isoflurane, which preserves the dependence of the caliber of cerebral vessels on the voltage of carbon dioxide, reduces the oxygen consumption of the brain, favorably influences the dynamics of cerebrospinal fluid, reducing its pressure. It must be borne in mind that during the maintenance of anesthesia, inhalational anesthetics are able to prolong the effect of nondepolarizing muscle relaxants. In particular, with enflurane anesthesia, the potentiation of the miorelaxing action of vecuronium is much stronger than with isoflurane and halothane. Therefore, doses of relaxants should be reduced in advance if strong inhalation anesthetics are used.
Contraindications
Common to all inhalation anesthetics is a contraindication is the lack of specific technical means for accurate dosage of the corresponding anesthetic (dosimeters, evaporators). A relative contraindication for many anesthetics is pronounced hypovolemia, the possibility of malignant hyperthermia and intracranial hypertension. In the rest, contraindications depend on the properties of inhalation and gaseous anesthetics.
Dinitrogen oxide and xenon are highly diffusive. The risk of filling closed cavities with gases restricts their use in patients with closed pneumothorax, air embolism, acute intestinal obstruction, neurosurgical operations (pneumocephaly), plastic operations on the tympanic membrane, etc. Diffusion of these anesthetics into the cuff of the endotracheal tube increases the pressure in it and can cause ischemia of the mucosal trachea. It is not recommended to use dinitrogen oxide in the postperfusion period and in operations in patients with heart defects with compromised hemodynamics due to cardiodepressive effect in this category of patients.
Do not show dinitrogen oxide and in patients with pulmonary hypertension, t. It increases pulmonary-vascular resistance. Do not use dinitrogen oxide in pregnant women to avoid a teratogenic effect.
Contraindication for the use of xenon is the need to apply hyperoxic mixtures (cardiac and pulmonary surgery).
For all other (except isoflurane) anesthetics, contraindications are conditions accompanied by increased intracranial pressure. Severe hypovolemia is a contraindication to the administration of isoflurane, sevoflurane, desflurane and enflurane because of their vasodilating effect. Halothane, sevoflurane, desflurane and enflurane are contraindicated in the risk of malignant hyperthermia.
Halothane causes depression of the myocardium, which limits its use in patients with severe heart disease. Do not use halothane in patients with dysfunction of the liver of an unknown genesis.
Kidney disease, epilepsy are additional contraindications for enflurane.
Tolerance and side effects
Dinitrogen oxide, oxidizing the irreversibly cobalt atom in vitamin Bi2, inhibits the activity of B12-dependent enzymes, such as methionine synthetase, necessary for myelin formation, and thymidelate synthetase, necessary for DNA synthesis. In addition, prolonged exposure of the dinitrogen oxide causes bone marrow depression (megaloblastic anemia) and even neurological deficits (peripheral neuropathy and funicular myelosis).
In connection with the fact that halothane is oxidized in the liver to its main metabolites - trifluoroacetic acid and bromide, postoperative liver dysfunctions are possible. Although Halothane hepatitis is rare (1 case for 35,000 ha-lotanovyh anesthesia), this anesthesiologist should remember.
It has been established that immune mechanisms play an important role in the hepatotoxic effect of halothane (eosinophilia, rash). Under the influence of trifluoroacetic acid, microsomal liver proteins play the role of trigger antigen, which triggers an autoimmune reaction.
Among the side effects of isofluren, moderate beta-adrenergic stimulation, an increase in blood flow in skeletal muscles, a reduction in total peripheral vascular resistance (OPSS), and AD (DE Morgan and MS Mikhail, 1998) should be mentioned. The depressive effect of isoflurane has on respiration, and to a somewhat greater extent than other inhalation anesthetics. Isoflurane reduces hepatic blood flow and diuresis.
Sevoflurane is degraded with the help of soda lime, which is filled with the absorber of the anesthesia and respiratory apparatus. At the same time, the concentration of the final product "A" increases if sevoflurane comes in contact with dry soda lime under closed loop conditions with a low gas flow. The risk of developing tubular necrosis of the kidneys increases significantly.
The toxic effect of an inhalation anesthetic depends on the percentage of metabolism of drugs: the more it is, the drugs are worse and more toxic.
Of the side effects of enflurane, mention should be made of the inhibition of myocardial contractility, a decrease in blood pressure and oxygen consumption, an increase in the heart rate (HR) and OPSS. In addition, enflurane sensitizes the myocardium to catecholamines, which should be borne in mind and do not apply epinephrine at a dose of 4.5 mcg / kg. Of the other side effects, we point out the respiratory depression when feeding 1 MAK LS-pC02 increases to 60 mm Hg with independent breathing. Art. To eliminate intracranial hypertension caused by enflurane, hyperventilation should not be used, especially if a high concentration of drugs is given, since an epileptiform fit can develop.
Side effects of anesthesia with xenon are observed in individuals who have a predilection for alcohol. In the initial period of anesthesia, they have pronounced psychomotor activity, leveled by the introduction of sedatives. In addition, there may be a syndrome of diffusion hypoxia due to the rapid elimination of xenon and the filling of the alveolar space. To prevent this phenomenon, it is necessary to ventilate the lungs of the patient with oxygen after turning off xenon for 4-5 minutes.
In clinical doses, halothane can cause depression of the myocardium, especially in patients with diseases of the cardiovascular system.
Interaction
During the maintenance of anesthesia, inhalation anesthetics are able to prolong the effect of nondepolarizing muscle relaxants, significantly reducing their consumption.
Because of weak anesthetic properties, dinitrogen oxide is usually used in combination with other inhalation anesthetics. This combination makes it possible to reduce the concentration of the second anesthetic in the respiratory mixture. Widely known and popular combinations of dinitrogen oxide with halothane, isoflurane, ether, cyclopropane. To increase the analgesic effect, dinitrogen oxide is combined with fentanyl and other anesthetics. An anesthetist should also be aware of another phenomenon where the use of a high concentration of one gas (eg, dinitrogen oxide) facilitates an increase in the alveolar concentration of another anesthetic (eg, halothane). This phenomenon is called the secondary gas effect. This increases ventilation (especially gas flow in the trachea) and the concentration of anesthetic at the level of the alveoli.
In connection with the fact that many anesthetists use combined methods of inhalation anesthesia, when vapor-like drugs are combined with dinitrogen oxide, it is important to know the hemodynamic effects of these combinations.
In particular, when dinitrogen oxide is added to halothane, cardiac output decreases, in response, the sympathoadrenal system is activated, leading to an increase in vascular resistance and an increase in blood pressure. When adding dinitrogen oxide to enflurane, a small or insignificant decrease in blood pressure and cardiac output occurs. Dinitrogen oxide in combination with isoflurane or desflurane at the level of MAK anesthetics leads to a certain increase in blood pressure, associated mainly with an increase in OPSS.
Dinitrogen oxide in combination with isoflurane significantly increases coronary blood flow on the background of a significant decrease in oxygen consumption. This indicates a violation of the mechanism of autoregulation of coronary blood flow. A similar picture is observed with the addition of dinitrogen oxide to enflurane.
Halothane combined with beta-blockers and calcium antagonists increases myocardial depression. Caution is necessary to combine the use of monoamine oxidase (MAO) inhibitors and tricyclic antidepressants with halothane due to the development of unstable blood pressure and arrhythmias. Dangerous combination of halothane with aminophylline due to the occurrence of severe ventricular arrhythmias.
Isoflurane is well combined with dinitrogen oxide and analgesics (fentanyl, remifentanil). Sevoflurane goes well with analgesics. It does not sensitize the myocardium to the arrhythmogenic effect of catecholamines. When interacting with soda lime (CO2 absorber), sevoflurane decomposes to form a nephrotoxic metabolite (compound A-olefin). This compound accumulates at a high temperature of respiratory gases (low-flow anesthesia), and therefore it is not recommended to use a fresh gas flow of less than 2 liters per minute.
Unlike some other drugs, desflurane does not cause myocardial sensitization to the arrhythmogenic effect of catecholamines (epinephrine can be used up to 4.5 μg / kg).
A good interaction with analgesics, muscle relaxants, neuroleptics, sedative drugs and inhalation anesthetics is also xenon. These agents potentiate the effect of the latter.
Attention!
To simplify the perception of information, this instruction for use of the drug "Inhalation anesthetics" translated and presented in a special form on the basis of the official instructions for medical use of the drug. Before use read the annotation that came directly to medicines.
Description provided for informational purposes and is not a guide to self-healing. The need for this drug, the purpose of the treatment regimen, methods and dose of the drug is determined solely by the attending physician. Self-medication is dangerous for your health.