Synthesis, secretion and metabolism of thyroid hormones
Last reviewed: 19.10.2021
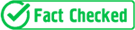
All iLive content is medically reviewed or fact checked to ensure as much factual accuracy as possible.
We have strict sourcing guidelines and only link to reputable media sites, academic research institutions and, whenever possible, medically peer reviewed studies. Note that the numbers in parentheses ([1], [2], etc.) are clickable links to these studies.
If you feel that any of our content is inaccurate, out-of-date, or otherwise questionable, please select it and press Ctrl + Enter.
The precursor of T 4 and T 3 is the amino acid L-tyrosine. The addition of iodine to the phenolic ring of tyrosine provides the formation of mono- or diiodotyrosines. If a second phenolic ring is attached to the tyrosine with the help of an ether bond, then tyronine is formed. To each of the two or at once to both phenol rings of tyronine, one or two iodine atoms in the meta position relative to the amino acid residue can attach. T4 is 3,5,3 ', 5'-tetraiodothyronine, and T 3 is 3,5,3'-triiodothyronine, i.e. Contains less than one iodine atom in the "outer" (amino acid-deprived) ring. When the iodine atom is removed from the "inner" ring, T 4 turns into 3,3'.5'-triiodothyronine or into reverse (reversible) T 3 (pT 3 ). Diiodothyronine can exist in three forms (3 ', 5'-T 2, 3,5-T 2 or 3,3'-T 2 ). When tetraiodo and triiodothyroacetic acids are cleaved from T 4 or T 3 amino groups, respectively. The considerable flexibility of the spatial structure of the thyroid hormone molecule, determined by the rotation of both tironin rings relative to the alanine part, plays an important role in the interaction of these hormones with the binding proteins of the blood plasma and cellular receptors.
The main natural source of iodine is marine products. The minimum daily requirement for iodine (in terms of iodide) for humans is about 80 μg, but in some areas where iodized salt is used for preventive purposes, iodide intake can reach 500 μg / day. The content of iodide is determined not only by the amount that comes from the gastrointestinal tract, but also by the "leakage" from the thyroid gland (normally about 100 μg / day), and by the peripheral deiodination of iodothyronines.
The thyroid gland has the ability to concentrate iodide from the blood plasma. Other tissues have a similar ability, for example, the gastric mucosa and salivary glands. The process of iodide transfer into the follicular epithelium is volatile, saturated and carried out in conjunction with the reverse transport of sodium by sodium-potassium adenosine triphosphatase (ATPase). The iodide transport system is not strictly specific and causes the delivery to the cell of a number of other anions (perchlorate, pertechnetate and thiocyanate), which are competitive inhibitors of the process of accumulation of iodide in the thyroid gland.
As already noted, in addition to iodine, a component of thyroid hormones is tyronine, which is formed in the interior of the protein molecule - thyroglobulin. Its synthesis occurs in thyroid cells. Thyreoglobulin accounts for 75% of all contained and 50% synthesized at any given time protein in the thyroid gland.
The iodide that enters the cell is oxidized and covalently attached to the tyrosine residues in the thyroglobulin molecule. Both oxidation and iodination of tyrosyl residues are catalyzed by the peroxidase present in the cell. Although the active form of iodine, the iodinated protein, is not exactly known, but before such iodination (i.e., the process of iodine addition) occurs, hydrogen peroxide must be formed. In all likelihood, it is produced by NADH-cytochrome B- or NADPH-cytochrome C-reductase. Both tyrosyl and monoiodo-thyro residues in the thyroglobulin molecule undergo iodization. This process is influenced by the nature of a number of located amino acids, as well as the tertiary conformation of thyroglobulin. Peroxidase is a membrane-bound enzyme complex, the prosthetic group of which forms heme. The hematinic grouping is absolutely necessary for the manifestation of enzyme activity.
The iodination of amino acids precedes their condensation, i.e., the formation of tyronine structures. The latter reaction requires the presence of oxygen and can be carried out through the intermediate formation of an active metabolite of iodotyrosine, for example pyruvic acid, which then joins the iodotyrosyl residue in the thyroglobulin composition. Regardless of which condensation mechanism exists, this reaction is also catalyzed by thyroid peroxidase.
Molecular weight of mature thyroglobulin is 660,000 daltons (sedimentation coefficient is 19). It apparently has a unique tertiary structure that condenses the condensation of iodotyrosyl residues. Indeed, the content of tyrosine in this protein differs little from that in other proteins, and iodination of tyrosyl residues can occur in any of them. However, the condensation reaction is carried out with a sufficiently high efficacy, probably only in thyroglobulin.
The content of iodine acids in native thyroglobulin depends on the availability of iodine. Normally thyroglobulin contains 0.5% of iodine consisting of 6 residues monoiodotyrosine (MIT), 4 - diiodotyrosine (DIT), 2 - T 4 and 0.2 - Ts protein molecule. Reverse T 3 and diiodothyronines are present in very small amounts. However, in terms of iodine deficiency these ratios are violated: increase ratio of MIT / DIT and T 3 / T 4, that are considered as active gormogeneza device in the thyroid to deficiency of iodine, as T 3 has a higher metabolic activity than T 4.
The entire process of the synthesis of thyroglobulin in the follicular cell of the thyroid gland is directed in one direction: from the basal membrane to the apical membrane and then to the colloidal space. The formation of free thyroid hormones and their entry into the blood presupposes the existence of an inverse process. The latter consists of a number of stages. Initially, the thyroglobulin contained in the colloid is captured by processes of microvilli of the apical membrane forming bubbles of pinocytosis. They move into the cytoplasm of the follicular cell, where they are called colloidal drops. In turn, they fuse with microsomes, forming phagolysosomes, and in their composition migrate to the basal cell membrane. During this process, proteolysis of thyroglobulin takes place, during which T 4 and T 3 are formed. The latter diffuse from the follicular cells into the blood. In the cell itself, partial deiodination of T 4 also occurs with the formation of T 3. Some of the iodothyrozines, iodine and a small amount of thyroglobulin also enter the bloodstream. The latter circumstance is essential for understanding the pathogenesis of autoimmune thyroid diseases, which are characterized by the presence of antibodies to thyroglobulin in the blood. In contrast to previous views, according to which the formation of such auto antibodies was associated with damage to thyroid tissue and the ingestion of thyroglobulin into the blood, it has now been shown that thyroglobulin is delivered there and is normal.
In the process of intracellular proteolysis of thyroglobulin, the cytoplasm of the follicular cell is penetrated not only by iodothyronines, but also by iodotyrosines contained in the protein in large amounts. However, unlike T 4 and T 3, they are rapidly deiodinated by the enzyme present in the microsomal fraction, with the formation of iodide. The greater part of the latter is exposed in the thyroid gland to a reutilization, but some of it still leaves the cell in the blood. Deiodination of iodotyrosines provides 2-3 times more iodide for a new synthesis of thyroid hormones than transportation of this anion from the plasma of the blood to the thyroid gland, and therefore plays a major role in maintaining the synthesis of iodine-ironons.
During the day, the thyroid gland produces about 80-100 μg of T 4. The half-life of this compound in the blood is 6-7 days. Every day, the body breaks down about 10% of the secreted T 4. The rate of its degradation, like T 3, depends on their binding to serum proteins and tissues. Under normal circumstances, more than 99.95% present in the blood T 4 and Ts 99.5% bound to plasma proteins. The latter act as a buffer of the level of free thyroid hormones and at the same time serve as a place for their storage. The distribution of T 4 and T 3 among the various binding proteins is affected by the pH and ionic composition of the plasma. In plasma, approximately 80% of T 4 is combined with thyroxine-binding globulin (TSH), 15% with thyroxine-binding prealbumin (TSPA), and the rest with serum albumin. TSH binds 90% of T 3, and TSPA binds 5% of this hormone. It is generally accepted that only an insignificant fraction of thyroid hormones that is not attached to proteins and is capable of diffusion through the cell membrane is metabolically active. In absolute figures, the amount of free T 4 in serum is about 2 ng%, and T 3 is 0.2 ng%. However, recently a number of data have been obtained on the possible metabolic activity and that part of the thyroid hormones that is associated with TPAA. It is not excluded that TSPA is an indispensable intermediary in the transfer of the hormonal signal from the blood to the cells.
TSG has a molecular weight of 63,000 daltons and is a glycoprotein synthesized in the liver. Its affinity for T 4 is about 10 times higher than for T 3. Carbohydrate component of TSG is represented by sialic acid and plays an essential role in the complexation of hormones. Hepatic production of TSH is stimulated by estrogens and is inhibited by androgens and large doses of glucocorticoids. In addition, there are congenital anomalies in the production of this protein, which can affect the total concentration of thyroid hormones in the blood serum.
The molecular weight of the TPAA is 55,000 daltons. At present, the complete primary structure of this protein is established. Its spatial configuration determines the existence of a channel molecule passing through the center, in which two identical binding sites are located. Complexion of T 4 with one of them sharply reduces the affinity of the second to the hormone. Like TSG, TSPA has a much greater affinity for T 4 than for T 3. It is interesting that other parts of TSPA are capable of binding a small (21 000) protein, specifically interacting with vitamin A. The attachment of this protein stabilizes the TSPA complex with T 4. It is important to note that severe non-thyroid diseases, as well as fasting, are accompanied by a rapid and significant drop in serum TSA level.
Serum albumin has the smallest of the listed proteins affinity for thyroid hormones. Since normal with albumin is associated with not more than 5% of the total amount of thyroid hormones present in the serum, a change in its level only has very little effect on the concentration of the latter.
As already noted, the combination of hormones with serum proteins not only prevents the biological effects of T 3 and T 4, but also significantly slows down the rate of their degradation. Up to 80% of T 4 is metabolized by monodeiodination. In the case of the detachment of the iodine atom at the 5'-th position, T3 is formed, which has much more biological activity; when iodine is cleaved at position 5, pT 3 is formed, whose biological activity is extremely insignificant. Monodeiodination of T 4 in one or another position is not an accidental process, but is regulated by a number of factors. However, in normal cases, deiodation in both positions usually proceeds at an equal rate. Small amounts of T 4 are deaminated and decarboxylated to form tetraiodothyroacetic acid, as well as conjugation with sulfuric and glucuronic acids (in the liver) followed by excretion of conjugates with bile.
Monodeiodination of T 4 outside the thyroid gland serves as the main source of T 3 in the body. This process provides almost 80% of 20-30 μg of T 3 formed per day. Thus the share of the secretion of T 3 by the thyroid gland is not more than 20% of its daily requirement. Vnetireoidnoe Ts formation of T 4 catalyzed T 4 5'-deiodinase. The enzyme is localized in the cellular microsomes and requires as a cofactor the reduced sulfhydryl groups. It is believed that the main transformation of T 4 into T3 occurs in the tissues of the liver and kidneys. T 3 is weaker than T 4, is bound to serum proteins, so it undergoes faster degradation. The period of its half-life in the blood is about 30 hours. It turns mainly into 3,3'-T 2 and 3,5-T 2; small amounts of triiodothyroacetic and triiodothyropropionic acids are formed, as well as conjugates with sulfuric and glucuronic acids. All these compounds are practically devoid of biological activity. The various diiodothyronines are then converted into monoiodothyronines and finally to free tyronine, which is found in the urine.
The concentration of various iodothyronines in the serum of a healthy person is, μg%: T 4 - 5-11; ng%: T 3 - 75-200, tetraiodothyroacetic acid - 100-150, pT 3 - 20-60, 3,3'-T 2 - 4-20, 3,5-T 2 - 2-10, triiodothyroacetic acid - 5-15, 3 ', 5'-T 2 - 2-10, 3-T, -2.5.