Synthesis, secretion and metabolism of hormones of the adrenal cortex
Last reviewed: 19.10.2021
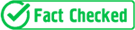
All iLive content is medically reviewed or fact checked to ensure as much factual accuracy as possible.
We have strict sourcing guidelines and only link to reputable media sites, academic research institutions and, whenever possible, medically peer reviewed studies. Note that the numbers in parentheses ([1], [2], etc.) are clickable links to these studies.
If you feel that any of our content is inaccurate, out-of-date, or otherwise questionable, please select it and press Ctrl + Enter.
Differences between the chemical structure of the main steroid compounds synthesized in the adrenal glands are reduced to the unequal saturation of carbon atoms and the presence of additional groupings. To designate steroid hormones, not only systematic chemical nomenclature (often very cumbersome) is used, but also trivial names.
The initial structure for the synthesis of steroid hormones is cholesterol. The amount of steroids produced depends on the activity of the enzymes that catalyze the individual stages of the corresponding transformations. These enzymes are localized in various cell fractions - mitochondria, microsomes and cytosol. Cholesterol, used for the synthesis of steroid hormones, is formed in the adrenal glands of acetate and partially enters the iron with molecules of low-density lipoproteins (LDL) or high-density (HDL) synthesized in the liver. Different sources of cholesterol in these cells are mobilized differently under different conditions. Thus, an increase in the production of steroid hormones in conditions of acute stimulation of ACTH is provided by the conversion of a small amount of free cholesterol formed as a result of the hydrolysis of these esters. Simultaneously, the synthesis of cholesterol from acetate also increases. With prolonged stimulation of the adrenal cortex, the synthesis of cholesterol, on the contrary, decreases, and its main source is plasma lipoproteins (against the background of an increase in the number of LDL receptors). With abetalipoproteinemia (lack of LDL), the adrenal glands react to ACTH with a lower cortisol release than normal.
In mitochondria, cholesterol is transformed into pregnenolone, which is the precursor of all steroid hormones of vertebrates. Its synthesis is a multi-stage process. It limits the rate of biosynthesis of adrenal steroids and is the object of regulation (from ACTH, angiotensin II and potassium, see below). In different areas of the adrenal cortex, pregnenolone undergoes various transformations. In the glomerular zone, it is mainly transformed into progesterone and further into 11-deoxy-corticosterone (DOC), and in the fascicle to 17a-oxypregnenolone, which serves as a precursor to cortisol, androgens and estrogens. 17a-hydroxyprogesterone is formed on the way of synthesis of cortisol from 17a-hydroxypregnonolone, which is subsequently hydroxylated by 21- and 11-beta-hydroxylases to 11-deoxy-cortisol (cortexolone, or compound S), and then (in mitochondria) to cortisol (hydrocortisone, or compound F).
The main product of the glomerular zone of the adrenal cortex is aldosterone, the synthesis route of which includes intermediate stages of progesterone, DOC, corticosterone (compound B) and 18-oxycorticosterone. The latter under the action of mitochondrial 18-hydroxysteroid dehydrogenase acquires an aldehyde grouping. This enzyme is present only in the glomerular zone. On the other hand, it lacks 17a-hydroxylase, which prevents the formation of cortisol in this zone. The MLC can be synthesized in all three zones of the cortex, but its greatest amount is produced in the beam zone.
Among the products of the secretion of the beam and reticular zones, there are also C-19 steroids with androgenic activity: dehydroepiandrosterone (DHEA), dehydroepiandrosterone sulfate (DHEA-C), androstenedione (and its 11beta analog) and testosterone. All of them are formed from 17a-oxypregnenolone. In quantitative terms, the main androgenes of the adrenal glands are DHEA and DHEA-C, which in iron can be converted into each other. Synthesis of DHEA occurs with the participation of 17a-hydroxylase, which is absent in the glomerular zone. Androgenic activity of adrenal steroids is mainly due to their ability to convert to testosterone. The adrenal glands themselves produce very little of this substance, as well as estrogens (estrone and estradiol). However, adrenal androgens can serve as a source of estrogens formed in subcutaneous adipose tissue, hair follicles, and mammary gland. In the fetal region of the adrenal cortex, 3beta-hydroxysteroid dehydrogenase activity is absent, and therefore the main products are DHEA and DHEA-C, which are converted into the placenta into estrogens, providing 90% of estriol and 50% estradiol and estrone in the maternal body.
Steroid hormones of the adrenal cortex are differently bound by plasma proteins. As for cortisol, 90-93% of the hormone present in the plasma is in a bound form. Approximately 80% of this binding is due to specific corticosteroid-binding globulin (transcortin), which has a high affinity for cortisol. A smaller amount of the hormone is connected with albumin and very little - with other plasma proteins.
Transcortin is synthesized in the liver. It is a glycosylated protein with a relative molecular weight of about 50,000, binding in a healthy person to 25 μg% cortisol. Therefore, at high concentrations of the hormone, the level of free cortisol will no longer be proportional to its total content in the plasma. Thus, with a total concentration of cortisol in plasma of 40 μg%, the concentration of free hormone (about 10 μg%) will be 10 times higher than with a total cortisol level of 10 μg%. As a rule, transcortin, due to its greatest affinity for cortisol, binds only to this steroid, but at the end of pregnancy as many as 25% of the transcortin-related steroid is represented by progesterone. The nature of the steroid in combination with transcortin can change with congenital adrenal hyperplasia, when the latter produce large amounts of corticosterone, progesterone, 11-deoxycortisol, MLC and 21-deoxycortisol. Most synthetic glucocorticoids are poorly linked to transcortin. Its level in the plasma is regulated by various (including hormonal) factors. So, estrogens increase the content of this protein. Thiroid hormones also have a similar property. An increase in the level of transcortin was observed in diabetes mellitus and a number of other diseases. For example, hepatic and renal (nephrosis) changes are accompanied by a decrease in the content of transcortin in plasma. Synthesis of transcortin can be inhibited by glucocorticoids. Genetically determined fluctuations in the level of this protein are usually not accompanied by clinical manifestations of hyper- or hypocorticism.
Unlike cortisol and a number of other steroids, aldosterone does not interact specifically with plasma proteins. It is only very weakly bound to albumin and transcortin, and also to red blood cells. Under physiological conditions, only about 50% of the total amount of the hormone is connected to plasma proteins, and 10% of it is associated with transcortin. Therefore, with an increase in the level of cortisol and complete saturation of transcortin, the level of free aldosterone may vary insignificantly. The association of aldosterone with transcortin is stronger than with other plasma proteins.
Adrenal androgens, with the exception of testosterone, are predominantly bound by albumin, and quite weakly. Testosterone is almost completely (98%) specifically interacts with testosterone-estradiol-binding globulin. The concentration of the latter in plasma increases under the influence of estrogens and thyroid hormones and decreases under the action of testosterone and STH.
Hydrophobic steroids are filtered by the kidneys, but almost entirely (95% cortisol and 86% aldosterone) are reabsorbed in tubules. For their isolation with urine, enzymatic transformations are necessary, increasing their solubility. They reduce mainly to the transition of ketone groups into carboxyl and C-21 groups into acidic forms. Hydroxyl groups are able to interact with glucuronic and sulfuric acids, which further increases the water solubility of steroids. Among the many tissues in which their metabolism occurs, the most important place is occupied by the liver, and in pregnancy - by the placenta. Part of the metabolized steroids enters the intestinal contents, from where they can be reabsorbed in an unchanged or modified form.
The disappearance of cortisol from the blood occurs with a half-period of 70-120 minutes (depending on the dose administered). During the day, about 70% of the labeled hormone falls into the urine; for 3 days with urine, 90% of such a hormone is excreted. About 3% is found in the stool. Unchanged cortisol is less than 1% of excreted labeled compounds. The first important stage of hormone degradation is the irreversible reduction of the double bond between the 4 th and 5 th carbon atoms. As a result of this reaction, 5 times more 5a-dihydrocortisol is formed than its 5beta-forms. Under the action of 3-hydroxysteroid-hydrogenase, these compounds rapidly transform into tetrahydrocortisol. Oxidation of the 11β-hydroxyl group of cortisol leads to the formation of cortisone. In principle, this transformation is reversible, but due to the smaller amount of cortisone produced by the adrenal glands, it is shifted toward the formation of this particular compound. The subsequent metabolism of cortisone occurs both in cortisol and goes through the stages of dihydro- and tetrahydroform. Therefore, the ratio between these two substances in urine is maintained for their metabolites. Cortisol, cortisone and their tetrahydroderivatives may undergo other transformations, including the formation of cortoles and cortholones, cortol and cortholonic acids (oxidation at the 21st position), and oxidation of the side chain at the 17th position. Bbeta-hydroxylated metabolites of cortisol and other steroids can also form. In children, as well as in a number of pathological conditions, this pathway of cortisol metabolism becomes of primary importance. 5-10% of metabolites of cortisol are C-19, 11-hydroxy and 17-ketosteroids.
The half-life of aldosterone in plasma does not exceed 15 minutes. It is almost completely extracted by the liver in one passage of blood, and less than 0.5% of the native hormone is found in the urine. Approximately 35% of aldosterone is excreted as tetrahydroldosterone glucuronide, and 20% is aldosterone glucuronide. This metabolite is called acid-labile, or 3-oxo-conjugate. Part of the hormone is found in the urine in the form of 21-deoxytetrahydroaldosterone, which is formed from the tetrahydrodosterone excreted with the bile by the action of the intestinal flora and is again absorbed into the blood.
For one passage of blood through the liver, more than 80% of androstenedione and only about 40% of testosterone are eliminated. In the urine, mainly androgen conjugates are found. A small fraction of them is excreted through the intestine. DHEA-C can be displayed unchanged. DHEA and DHEA-C are capable of further metabolism through hydroxylation at the 7th and 16th positions or the conversion of the 17-keto group to the 17-hydroxy group. DHEA is irreversibly transformed into androstenedione. The latter can be converted into testosterone (mainly outside the liver), as well as into androsterone and etiocholanolone. Further recovery of these steroids leads to the formation of androstanediol and etiocholandiol. Testosterone in target tissues is converted to 5a-dihydrotestosterone, which is irreversibly inactivated, turning into Z-androstanediol, or reversibly into 5a-androstenedione. Both these substances can be transformed into androsterone. Each of these metabolites is able to form glucuronides and sulfates. In men, testosterone and androstenedione disappear from the plasma 2-3 times faster than in women, which is probably explained by the effect of sex steroids on the level of testosterone-estradiol-binding protein in plasma.
Physiological effects of adrenal cortex hormones and the mechanism of their action
The compounds produced by the adrenal glands affect many metabolic processes and body functions. Already the names themselves - gluco- and mineralocorticoids - show that they perform important functions in the regulation of various aspects of metabolism.
An excess of glucocorticoids increases the formation of glycogen and the production of glucose by the liver and reduces the absorption and utilization of glucose by peripheral tissues. As a result, there is hyperglycemia and a decrease in glucose tolerance. In contrast, glucocorticoids deficiency decreases liver glucose production and increases insulin sensitivity, which can lead to hypoglycemia. The effects of glucocorticoids are opposite to that of insulin, the secretion of which increases in conditions of steroid hyperglycemia. This leads to normalization of the blood glucose level in the fasting blood, although a violation of tolerance to carbohydrates can persist. In conditions of diabetes mellitus, the excess of glucocorticoids aggravates the violation of glucose tolerance and increases the body's need for insulin. With addison's disease, less insulin is released in response to glucose intake (due to a small increase in blood sugar levels), so that the tendency to hypoglycemia softens and the fasting sugar level usually remains normal.
Stimulation of hepatic glucose production under the influence of glucocorticoids is explained by their effect on the processes of gluconeogenesis in the liver, the release of substrates of gluconeogenesis from peripheral tissues and the gluconeogenic effect of other hormones. Thus, in baseline adrenalectomized animals, basal gluconeogenesis persists, but its ability to increase under the action of glucagon or catecholamines is lost. In hungry or diabetic animals, adrenalectomy leads to a decrease in the intensity of gluconeogenesis, which is restored by the administration of cortisol.
Under the influence of glucocorticoids, virtually all stages of gluconeogenesis are activated. These steroids increase the overall protein synthesis in the liver with increasing the formation of a number of transaminases. However, the most important steps for glucocorticoids are the stages of gluconeogenesis, apparently after the reamination reactions, at the level of functioning of phosphoenolpyruvate carboxylase and glucose-6-phosphate dehydrogenase, whose activity in the presence of cortisol increases.
In muscles, fat and lymphoid tissues, steroids not only inhibit the synthesis of protein, but also accelerate its decay, which leads to the release of amino acids into the blood. In humans, the acute effect of glucocorticoids is manifested by a selective and pronounced increase in the content of amino acids in the plasma with a branched chain. With prolonged action of steroids, only the level of alanine increases in it. Against the background of fasting, the level of amino acids rises only briefly. The rapid effect of glucocorticoids is probably due to their anti-insulin action, and selective release of alanine (the main substrate of gluconeogenesis) is due to direct stimulation of the processes of transamination in tissues. Under the influence of glucocorticoids, the release of glycerin from adipose tissue (due to stimulation of lipolysis) and lactate from muscles also increases. Acceleration of lipolysis leads to increased intake of blood and free fatty acids, which, although they do not serve as direct substrates for gluconeogenesis, but, providing this process with energy, save other substrates that can be converted into glucose.
An important effect of glucocorticoids in the field of carbohydrate metabolism is the inhibition of glucose uptake and utilization by peripheral tissues (mainly fat and lymphoid). This effect can occur even earlier than stimulation of gluconeogenesis, so that after the administration of cortisol, glycemia rises even without increasing glucose production by the liver. There is also evidence of glucocorticoid stimulation of glucagon secretion and inhibition of insulin secretion.
The redistribution of fat in the body (deposition on the neck, face and trunk and disappearance of the limbs) observed in the Itenko-Cushing syndrome may be due to the unequal sensitivity of various fat stores to steroids and insulin. Glucocorticoids facilitate the lipolytic action of other hormones (growth hormone, catecholamines). The effect of glucocorticoids on lipolysis is mediated by inhibition of glucose uptake and metabolism in adipose tissue. As a result, it reduces the amount of glycerin necessary for the re-esterification of fatty acids, and more free fatty acids enter the bloodstream. The latter causes a tendency to ketosis. In addition, glucocorticoids can directly stimulate ketogenesis in the liver, which is especially pronounced in conditions of insulin deficiency.
For individual tissues, the effect of glucocorticoids on the synthesis of specific RNAs and proteins has been studied in detail. However, they also have a more general effect in the body, which is to stimulate the synthesis of RNA and protein in the liver, its inhibition and stimulation of decay in peripheral tissues such as muscles, skin, fat and lymphoid tissue, fibroblasts, but not the brain or heart.
Their direct effects on the cells of the body glucocorticoids, like other steroid compounds, exert through the initial interaction with the cytoplasmic receptors. They have a molecular mass of about 90,000 daltons and are asymmetric and possibly phosphorylated proteins. In each target cell, there are from 5000 to 100,000 cytoplasmic receptors of glucocorticoids. The binding affinity of these proteins with the hormone practically coincides with the concentration of free cortisol in the plasma. This means that the saturation of the receptors normally ranges from 10 to 70%. There is a direct correlation between the binding of steroids by cytoplasmic receptors and the glucocorticoid activity of hormones.
Interaction with the hormone causes a change in the conformation (activation) of the receptors, as a result of which 50-70% of the hormone receptor complexes bind to certain areas of nuclear chromatin (acceptors) containing DNA and, possibly, some nuclear proteins. The acceptor sites are present in the cell in such a large quantity that they are never completely saturated with hormone receptor complexes. Some part of the acceptors interacting with these complexes generates a signal that leads to an acceleration of the transcription of specific genes with a subsequent increase in the level of mRNA in the cytoplasm and an increase in the synthesis of proteins encoded by them. Such proteins may be enzymes (eg, those taking part in gluconeogenesis processes), which will determine specific responses to the hormone. In some cases, glucocorticoids reduce the level of specific mRNAs (eg, those that code for the synthesis of ACTH and beta endorphin). The presence of glucocorticoid receptors in most tissues distinguishes these hormones from steroids of other classes, the tissue representation of the receptors to which is much more limited. The concentration of glucocorticoid receptors in the cell limits the magnitude of the response to these steroids, which distinguishes them from other classes of hormones (polypeptide, catecholamines) for which there is a "redundancy" of the surface receptors on the cell membrane. Since the glucocorticoid receptors in different cells seem to be the same, and the reactions to cortisol depend on the type of cells, the expression of a particular gene under the action of a hormone is determined by other factors.
Recently, data on the possible effects of glucocorticoids not only through gene transcription mechanisms but also, for example, by modifying membrane processes, have accumulated, but the biological significance of such effects remains unclear. There are also reports of the heterogeneity of glucocorticoid-binding cellular proteins, but whether they are all true receptors is unknown. Although steroids belonging to other classes can interact with glucocorticoid receptors, their affinity for these receptors is generally less than for specific cell proteins mediating other, in particular mineralocorticoid, effects.
Mineralocorticoids (aldosterone, cortisol and sometimes DOC) regulate ion homeostasis, affecting the kidneys, intestines, salivary and sweat glands. Their direct action on the vascular endothelium, heart and brain is also possible. However, in any case, the number of tissues sensitive to mineralocorticoids in the body is much less than the number of tissues that react to glucocorticoids.
The most important of the currently known target organs of mineralocorticoids are the kidneys. Most of the effects of these steroids are located in the collecting canals of the cortex, where they contribute to an increase in sodium reabsorption, as well as the secretion of potassium and hydrogen (ammonium). These actions of mineralocorticoids occur 0.5-2 hours after their administration, accompanied by activation of the synthesis of RNA and protein and persist for 4-8 hours. With a deficiency of mineralocorticoids, the body develops sodium loss, potassium retention and metabolic acidosis. Excess hormones cause opposite shifts. Under the action of aldosterone, only a part of sodium filtered by the kidneys is reabsorbed, so this hormone effect manifests itself weaker in conditions of salt load. Moreover, even with the normal consumption of sodium in conditions of excess aldosterone, the phenomenon of escaping from its effect arises: the reabsorption of sodium in the proximal tubule canals decreases and finally its excretion comes in accordance with the consumption. The presence of this phenomenon can explain the absence of edema with a chronic excess of aldosterone. However, with edemas of cardiac, hepatic or renal origin, the body's ability to "escape" from the action of mineralocorticoids is lost, and the secondary hyperaldosteronism developing under such conditions aggravates the fluid retention.
With respect to the secretion of potassium by the kidney canals, the phenomenon of escape is absent. This effect of aldosterone is largely dependent on sodium intake and becomes evident only in conditions of sufficient intake of the latter into the distal renal tubules, where the effect of mineralocorticoids on its reabsorption is manifested. Thus, in patients with reduced glomerular filtration rate and increased sodium reabsorption in the proximal renal tubules (heart failure, nephrosis, cirrhosis), the potassium -uretic effect of aldosterone is practically absent.
Mineralocorticoids also increase the excretion of magnesium and calcium in the urine. These effects, in turn, are associated with the action of hormones on the renal dynamics of sodium.
Important effects of mineralocorticoids in the field of hemodynamics (in particular, changes in blood pressure) are largely mediated by their renal action.
The mechanism of cellular effects of aldosterone - as a whole as in other steroid hormones. In target cells, there are cytosolic receptors of mineralocorticoids. Their affinity for aldosterone and PKD far exceeds the affinity for cortisol. After interaction with the steroid penetrated into the cell, hormone-binding complexes bind to nuclear chromatin, increasing the transcription of certain genes with the formation of specific mRNA. The subsequent reactions due to the synthesis of specific proteins are probably due to an increase in the number of sodium channels on the apical surface of the cell. In addition, the ratio of NDD-H / NAD and the activity of a number of mitochondrial enzymes (citrate synthetase, glutamate dehydrogenase, malate dehydrogenase and glutamate oxalacetate transaminase), which participate in the generation of the biological energy necessary for the functioning of sodium pumps (on the serous surface of the distal renal tubules) under the influence of aldosterone in the kidneys, . The effect of aldosterone on phospholipase and acyltransferase activity is also not excluded, as a result of which the phospholipid composition of the cell membrane and ion transport change. The mechanism of action of mineralocorticoids on the secretion of potassium and the hydrogen ion in the kidneys is less studied.
Effects and mechanism of action of adrenal androgens and estrogens are discussed in the chapters on sex steroid.
Regulation of the secretion of hormones by the adrenal cortex
The production of adrenal glucocorticoids and androgens is controlled by the hypothalamic-pituitary system, whereas aldosterone production is predominantly by the renin-angiotensin system and potassium ions.
In the hypothalamus, corticoliberin is produced, which enters through the portal vessels into the anterior pituitary gland, where it stimulates the production of ACTH. Vasopressin also has a similar activity. ACTH secretion is regulated by three mechanisms: endogenous rhythm of corticoliberin release, stressor release and negative feedback mechanism, realized mainly by cortisol.
ACTH causes rapid and abrupt shifts in the cortical layer of the adrenal glands. Blood flow in the gland and synthesis of cortisol increase only 2-3 minutes after the introduction of ACTH. In a few hours, the mass of the adrenal glands can double. Lipids disappear from the cells of the bundle and reticular zones. Gradually, the boundary between these zones is smoothed out. The cells of the bundle zone are likened to the cells of the reticular, which creates the impression of a sharp expansion of the latter. Long stimulation of ACTH causes both hypertrophy and hyperplasia of the adrenal cortex.
An increase in the synthesis of glucocorticoids (cortisol) is due to the acceleration of the conversion of cholesterol to pregnenolone in the beam and reticular zones. Probably, other stages of the biosynthesis of cortisol, as well as its excretion into the blood, are activated. At the same time, small amounts of intermediate cortisol biosynthesis products enter the bloodstream. With a longer stimulation of the cortex, the formation of total protein and RNA increases, which leads to hypertrophy of the gland. Already after 2 days you can register an increase in the amount of DNA in it, which continues to grow. In the case of adrenal atrophy (as with ACTH decrease), the latter react to endogenous ACTH much more slowly: stimulation of steroidogenesis occurs almost every other day and reaches a maximum only by the 3rd day after the initiation of replacement therapy, the absolute magnitude of the reaction being reduced.
On the membranes of the adrenal cells, the sites linking ACTH with different affinities have been found. The number of these sites (receptors) decreases at high and increases with a low concentration of ACTH ("decreasing regulation"). Nevertheless, the general sensitivity of the adrenal glands to ACTH in conditions of high content does not only not decrease, but, on the contrary, increases. It is not excluded that ACTH under such conditions stimulates the appearance of some other factors, the effect of which on the adrenal gland "overcomes" the effect of decreasing regulation. Like other peptide hormones, ACTH activates adenylate cyclase in target cells, which is accompanied by the phosphorylation of a number of proteins. However, the sterogenic effect of ACTH is possibly mediated by other mechanisms, for example, by the potassium-dependent activation of the adrenal phospholipase A 2. Whatever it was, but under the influence of ACTH, the activity of esterase increases, releasing cholesterol from its esters, and the synthesis of cholesterol esters is inhibited. The seizure of lipoproteins by adrenal cells also increases. Then free cholesterol on the carrier protein enters the mitochondria, where it turns into pregnenolone. The effect of ACTH on cholesterol metabolism enzymes does not require the activation of protein synthesis. Under the influence of ACTH, the conversion of cholesterol to pregnenolone is apparently accelerated. This effect is no longer manifested in conditions of inhibition of protein synthesis. The mechanism of trophic influence of ACTH is unclear. Although the hypertrophy of one of the adrenals after removal of the second is probably related to the activity of the pituitary gland, but a specific antiserum to ACTH does not prevent such hypertrophy. Moreover, the introduction of ACTH itself during this period even reduces the content of DNA in the hypertrophied gland. In vitro ACTH also inhibits the growth of adrenal cells.
There is a circadian rhythm of the secretion of steroids. The level of cortisol in the plasma begins to increase after several hours after the onset of the night sleep, reaches a maximum shortly after waking up and falls in the morning hours. After noon and until the evening, the cortisol content remains very low. These episodes are superimposed with episodic "bursts" of cortisol level, which occur at different intervals - from 40 minutes to 8 hours or more. These emissions account for about 80% of all adrenal cortisol secreted. They are synchronized with ACTH peaks in the plasma and, apparently, with the release of hypothalamic corticoliberin. Regimes of nutrition and sleep play an important role in determining the periodic activity of the hypothalamic-pituitary-adrenal system. Under the influence of various pharmacological agents, as well as in pathological conditions, the circadian rhythm of ACTH and cortisol secretion is disrupted.
Significant place in the regulation of the activity of the system as a whole takes the mechanism of negative feedback between glucocorticoids and the formation of ACTH. The first inhibit the secretion of corticoliberin and ACTH. Under conditions of stress, the release of ACTH in adrenalectomized individuals is much greater than in intact ones, whereas exogenous administration of glucocorticoids significantly limits the increase in plasma ACTH concentration. Even in the absence of stress, adrenal insufficiency is accompanied by a 10-20-fold increase in the level of ACTH. Reduction of the latter in humans is observed only 15 minutes after the administration of glucocorticoids. This early inhibitory effect depends on the rate of increase in the concentration of the latter and is mediated, probably, by their effect on the membrane of the pituitary. The later inhibition of pituitary activity depends mainly on the dose (and not the rate) of the injected steroids and manifests itself only under conditions of intact synthesis of RNA and protein in corticotrophs. There are data indicating the possibility of mediating early and late inhibitory effects of glucocorticoids by different receptors. The relative role of oppression of corticoliberin secretion and ACTH itself in the feedback mechanism requires further clarification.
Adrenal production of mineralocorticoids is regulated by other factors, among which the most important is the renin-angiotensin system. The secretion of renin by the kidneys is controlled primarily by the concentration of the chloride ion in the liquid surrounding the juxtaglomerular cells, as well as by pressure in the renal vessels and beta-adrenergic substances. Renin catalyzes the conversion of angiotensinogen to decapeptide angiotensin I, which, splitting, forms octapeptide angiotensin II. In some species, the latter undergoes further transformations with the release of heptapeptide angiotensin III, which is also capable of stimulating the production of aldosterone and other mineralocorticoids (MRL, 18-oxycorticosterone and 18-oxideoxy corticosterone). In human plasma, the level of angiotensin III is no more than 20% of the level of angiotensin P. Both stimulate not only the conversion of cholesterol to pregnenolone, but also corticosterone into 18-hydroxycorticosterone and aldosterone. It is believed that the early effects of angiotensin are due to stimulation mainly of the initial stage of the synthesis of aldosterone, whereas in the mechanism of long-term effects of angiotensin, its effect on subsequent stages of the synthesis of this steroid plays a big role. On the surface of cells of the glomerular zone, there are angiotensin receptors. Interestingly, in the presence of excess angiotensin II, the number of these receptors does not decrease, but on the contrary, it increases. Potassium ions have a similar effect. Unlike ACTH, angiotensin II does not activate adrenal cyclase of the adrenal glands. Its action depends on the concentration of calcium and is mediated, probably, by the redistribution of this ion between the extra- and intracellular medium. A role in mediating the effect of angiotensin on the adrenals can be played by the synthesis of prostaglandins. Thus, prostaglandins of the E series (their serum level after the introduction of angiotensin II increases), in contrast to PIT, are able to stimulate the secretion of aldosterone, and inhibitors of the synthesis of prostaglandins (indomethacin) reduce the secretion of aldosterone and its reaction to angiotensin II. The latter also has a trophic effect on the glomerular zone of the adrenal cortex.
Increasing the level of potassium in the plasma also stimulates the production of aldosterone, and the adrenal glands are highly sensitive to potassium. Thus, a change in its concentration of only 0.1 meq / l, even within physiological fluctuations, affects the rate of aldosterone secretion. The potassium effect does not depend on sodium or angiotensin II. In the absence of kidneys, it is probably potassium that plays a major role in the regulation of aldosterone production. On the function of the beam zone of the adrenal cortex, its ions do not influence. Directly acting on the production of aldosterone, potassium at the same time reduces the production of renin by the kidneys (and accordingly the concentration of angiotensin II). However, the direct effect of its ions usually turns out to be stronger than the counter-regulator effect mediated by a decrease in renin. Potassium stimulates both early (transformation of cholesterol into pregnenolone), and late (changes in corticosterone or MTCT in aldosterone) stages of biosynthesis of mineralocorticoids. Under hyperkalemia, the ratio of concentrations of 18-oxycorticosterone / aldosterone in plasma increases. The effects of potassium on the adrenal cortex, like the action of angiotensin II, depend heavily on the presence of potassium ions.
The secretion of aldosterone is controlled by the level of sodium in the serum. The salt load reduces the production of this steroid. To a large extent this effect is mediated by the effect of sodium chloride on the release of renin. However, direct action of sodium ions on aldosterone synthesis is also possible, but it requires very sharp differences in cation concentration and has less physiological significance.
Neither hypophysectomy nor suppression of ACTH secretion with dexamethasone does not affect the production of aldosterone. However, in the conditions of prolonged hypopituitarism or isolated deficiency of ACTH, the reaction of aldosterone to the restriction of sodium in the diet may decrease or even completely disappear. In humans, the introduction of ACTH transiently increases the secretion of aldosterone. It is interesting that a decrease in its level in patients with ACTH deficiency is not manifested in glucocorticoid therapy, although glucocorticoids themselves can inhibit steroidogenesis in the glomerular zone. A definite role in the regulation of aldosterone production appears to be due to dopamine, since its agonists (bromocriptine) inhibit the steroid response to angiotensin II and ACTH, and antagonists (metoclopramide) increase the level of aldosterone in plasma.
As for the secretion of cortisol, circadian and episodic fluctuations are characteristic for plasma aldosterone levels, although they are much less pronounced. The concentration of aldosterone is highest after midnight - up to 8-9 hours and the lowest from 16 to 23 hours. The frequency of cortisol secretion does not affect the rhythm of aldosterone release.
Unlike the latter, the production of androgens by the adrenal glands is regulated mainly by ACTH, although other factors may participate in regulation. Thus, in the prepubescent period there is a disproportionately high secretion of adrenal androgens (in relation to cortisol), called adrenarche. However, it is possible that this is due not so much to the different regulation of the production of glucocorticoids and androgens, but rather to the spontaneous rearrangement of steroid biosynthesis pathways in the adrenal glands during this period. In women, the androgen level in the plasma depends on the phase of the menstrual cycle and is largely determined by the activity of the ovaries. However, in the follicular phase, almost 70% of testosterone, 50% of dihydrotestosterone, 55% of androstenedione, 80% of DHEA, and 96% of DHEA-C are responsible for the proportion of adrenal steroids in the total androgen concentration in the plasma. In the middle of the cycle, the adrenal contribution to the total androgen concentration drops to 40% for testosterone and 30% for androstenedione. In men, the adrenal glands play a very minor role in creating the total androgen concentration in the plasma.
Adrenal production of mineralocorticoids is regulated by other factors, among which the most important is the renin-angiotensin system. The secretion of renin by the kidneys is controlled primarily by the concentration of the chloride ion in the liquid surrounding the juxtaglomerular cells, as well as by pressure in the renal vessels and beta-adrenergic substances. Renin catalyzes the conversion of angiotensinogen to decapeptide angiotensin I, which, splitting, forms octapeptide angiotensin II. In some species, the latter undergoes further transformations with the release of heptapeptide angiotensin III, which is also capable of stimulating the production of aldosterone and other mineralocorticoids (MRL, 18-oxycorticosterone and 18-oxideoxy corticosterone). In human plasma, the level of angiotensin III is no more than 20% of the level of angiotensin P. Both stimulate not only the conversion of cholesterol to pregnenolone, but also corticosterone into 18-hydroxycorticosterone and aldosterone. It is believed that the early effects of angiotensin are due to stimulation mainly of the initial stage of the synthesis of aldosterone, whereas in the mechanism of long-term effects of angiotensin, its effect on subsequent stages of the synthesis of this steroid plays a big role. On the surface of cells of the glomerular zone, there are angiotensin receptors. Interestingly, in the presence of excess angiotensin II, the number of these receptors does not decrease, but on the contrary, it increases. Potassium ions have a similar effect. Unlike ACTH, angiotensin II does not activate adrenal cyclase of the adrenal glands. Its action depends on the concentration of calcium and is mediated, probably, by the redistribution of this ion between the extra- and intracellular medium. A role in mediating the effect of angiotensin on the adrenals can be played by the synthesis of prostaglandins. Thus, prostaglandins of the E series (their serum level after the introduction of angiotensin II increases), in contrast to PIT, are able to stimulate the secretion of aldosterone, and inhibitors of the synthesis of prostaglandins (indomethacin) reduce the secretion of aldosterone and its reaction to angiotensin II. The latter also has a trophic effect on the glomerular zone of the adrenal cortex.
Increasing the level of potassium in the plasma also stimulates the production of aldosterone, and the adrenal glands are highly sensitive to potassium. Thus, a change in its concentration of only 0.1 meq / l, even within physiological fluctuations, affects the rate of aldosterone secretion. The potassium effect does not depend on sodium or angiotensin II. In the absence of kidneys, it is probably potassium that plays a major role in the regulation of aldosterone production. On the function of the beam zone of the adrenal cortex, its ions do not influence. Directly acting on the production of aldosterone, potassium at the same time reduces the production of renin by the kidneys (and accordingly the concentration of angiotensin II). However, the direct effect of its ions usually turns out to be stronger than the counter-regulator effect mediated by a decrease in renin. Potassium stimulates both early (transformation of cholesterol into pregnenolone), and late (changes in corticosterone or MTCT in aldosterone) stages of biosynthesis of mineralocorticoids. Under hyperkalemia, the ratio of concentrations of 18-oxycorticosterone / aldosterone in plasma increases. The effects of potassium on the adrenal cortex, like the action of angiotensin II, depend heavily on the presence of potassium ions.
The secretion of aldosterone is controlled by the level of sodium in the serum. The salt load reduces the production of this steroid. To a large extent this effect is mediated by the effect of sodium chloride on the release of renin. However, direct action of sodium ions on aldosterone synthesis is also possible, but it requires very sharp differences in cation concentration and has less physiological significance.
Neither hypophysectomy nor suppression of ACTH secretion with dexamethasone does not affect the production of aldosterone. However, in the conditions of prolonged hypopituitarism or isolated deficiency of ACTH, the reaction of aldosterone to the restriction of sodium in the diet may decrease or even completely disappear. In humans, the introduction of ACTH transiently increases the secretion of aldosterone. It is interesting that a decrease in its level in patients with ACTH deficiency is not manifested in glucocorticoid therapy, although glucocorticoids themselves can inhibit steroidogenesis in the glomerular zone. A definite role in the regulation of aldosterone production appears to be due to dopamine, since its agonists (bromocriptine) inhibit the steroid response to angiotensin II and ACTH, and antagonists (metoclopramide) increase the level of aldosterone in plasma.
As for the secretion of cortisol, circadian and episodic fluctuations are characteristic for plasma aldosterone levels, although they are much less pronounced. The concentration of aldosterone is highest after midnight - up to 8-9 hours and the lowest from 16 to 23 hours. The frequency of cortisol secretion does not affect the rhythm of aldosterone release.
Unlike the latter, the production of androgens by the adrenal glands is regulated mainly by ACTH, although other factors may participate in regulation. Thus, in the prepubescent period there is a disproportionately high secretion of adrenal androgens (in relation to cortisol), called adrenarche. However, it is possible that this is due not so much to the different regulation of the production of glucocorticoids and androgens, but rather to the spontaneous rearrangement of steroid biosynthesis pathways in the adrenal glands during this period. In women, the androgen level in the plasma depends on the phase of the menstrual cycle and is largely determined by the activity of the ovaries. However, in the follicular phase, almost 70% of testosterone, 50% of dihydrotestosterone, 55% of androstenedione, 80% of DHEA, and 96% of DHEA-C are responsible for the proportion of adrenal steroids in the total androgen concentration in the plasma. In the middle of the cycle, the adrenal contribution to the total androgen concentration drops to 40% for testosterone and 30% for androstenedione. In men, the adrenal glands play a very minor role in creating the total androgen concentration in the plasma.