Medical expert of the article
New publications
Memory: neurochemical mechanisms of memory
Last reviewed: 23.04.2024
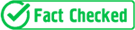
All iLive content is medically reviewed or fact checked to ensure as much factual accuracy as possible.
We have strict sourcing guidelines and only link to reputable media sites, academic research institutions and, whenever possible, medically peer reviewed studies. Note that the numbers in parentheses ([1], [2], etc.) are clickable links to these studies.
If you feel that any of our content is inaccurate, out-of-date, or otherwise questionable, please select it and press Ctrl + Enter.
Although the molecular mechanisms of the functioning of a single nerve cell have been studied in many of their manifestations and the principles of organization of interneuronal connections have been formulated, it is still unclear how the molecular properties of neurons provide storage, reproduction and analysis of information-memory.
The fact that acquired knowledge (like moral principles) is not inherited, and new generations have to be taught again, allows us to consider that learning is the process of creating new inter-neural connections and remembering information is provided by the ability of the brain to reproduce these links as necessary (activate their). However, modern neurochemistry is not yet able to provide a consistent theory describing how the analysis of the factors of the external world takes place in the living brain. One can only outline the problems that scientists of different areas of neurobiology are working on intensively.
Almost all kinds of animals are capable of analyzing changes in the external environment to a greater or lesser degree and responding adequately to them. In this case, the repeated reaction of the body to external effects is often different than in the first collision. This observation shows that living systems have the ability to learn. They have memory that preserves the personal experience of the animal, which forms behavioral reactions and can differ from the experience of other individuals.
Biological memory is diverse. It is inherent not only in brain cells. Memory of the immune system, for example, for a long time (often for life) keeps information about the once-in-the-body foreign antigen. When you re-meet, the immune system triggers an antibody reaction that allows you to quickly and effectively defeat the infection. However, the immune system "knows" how to respond to a known factor, and when encountering an unknown agent, it must develop an behavior strategy anew. The nervous system, unlike the immune system, can be trained to create a strategy of behavior in the new circumstances, based on the "life experience", which makes it possible to develop an effective response to an unknown stimulus.
The main questions to be answered in the study of molecular mechanisms of memory are: what metabolic changes occur in neurons when they meet with an external stimulus, which allow the stored information to be preserved for a certain (sometimes long) time; in what form the received information is stored; how is it analyzed?
In the process of active learning, occurring at an early age, there are changes in the structure of neurons, the density of synaptic contacts increases, the ratio of glial and nerve cells increases. It is difficult to distinguish the process of maturation of the brain and structural changes, which are molecular carriers of memory. However, it is clear that for the full development of intelligence it is necessary to solve the tasks posed by the external environment (recall the phenomenon of Mowgli or the problems of adaptation to life in nature of animals grown in captivity).
In the last quarter of the XX century. Attempts were made to study in detail the morphological features of the brain of A. Einstein. However, the result was rather disappointing - no features that distinguish it from the average modern brain were revealed. The only exception was a certain (insignificant) excess of the ratio of glial and nerve cells. Does this mean that the molecular processes of memory leave no visible traces in the nerve cells?
On the other hand, it has long been established that DNA synthesis inhibitors do not affect memory, while inhibitors of transcription and translation impair memory processes. Does this mean that certain proteins in brain neurons are memory carriers?
The organization of the brain is such that the main functions associated with the perception of external signals and reactions to them (for example, with motor reaction) are localized in certain parts of the cerebral cortex. Then the development of the acquired reactions (conditioned reflexes) should be a "closure of the bonds" between the corresponding centers of the cortex. The experimental damage to this center must destroy the memory of this reflex.
However, experimental neurophysiology has accumulated a lot of evidence that the memory of acquired skills is distributed to different parts of the brain, and not concentrated only in the area responsible for the function in question. Experiments with partial disturbance of the cortex in rats trained to orientate themselves in the maze showed that the time required to restore the disrupted skill is proportional to the volume of destruction and does not depend on its localization.
Probably, the development of behavior in the labyrinth involves the analysis of a whole set of factors (olfactory, taste, visual), and the brain regions responsible for this analysis can be located in different areas of the brain. Thus, although for each component of the behavioral response there is a certain part of the brain, the general reaction is realized when they interact. Nevertheless, in the brain, departments have been found whose function is directly related to memory processes. It is a hippocampus and an amygdaloid complex, as well as nuclei of the midline of the thalamus.
A set of changes in the CNS, associated with the fixation of information (image, type of behavior, etc.), neuroscientists are called an engram. Modern ideas about the molecular mechanisms of memory suggest that the involvement of individual brain structures in the process of memorizing and storing information does not consist of storing specific engrams, but in regulating the creation and functioning of neural networks that capture, record and reproduce information.
In general, the data accumulated in the study of behavioral reflexes and electrical activity of the brain indicate that both behavioral and emotional manifestations of vital activity are not localized in a certain group of neurons of the brain, but are expressed in a change in the interactions of a large number of nerve cells that reflect the functioning of the entire brain as of an integrated system.
To describe the flow of the process of remembering new information over time, the terms short-term memory and long-term memory are often used. In the short-term memory, information can be stored from fractions of a second to tens of minutes, while in long-term memory, information is sometimes contained throughout life. To convert the first type of memory into a second one, a so-called consolidation process is needed. Sometimes it is allocated to a separate stage of intermediate memory. However, all these terms, probably reflecting the obvious processes, are not yet filled with real biochemical data.
Types of memory and their modulation (by: Ashmarin, 1999)
Types of memory |
Inhibitors, effects |
Short-term memory |
Electroshock, cholinolytics (atropine, scopolamine), galanin, US1 (introduction to specific parts of the brain) |
Intermediate memory (consolidation) |
Inhibitors of energy metabolism, uabain, hypoxia, inhibitors of the synthesis of RNA and proteins (anisomycin, cycloheximide, puromycin, actinomycin O, RNase), antibodies to neurospecific proteins (vasopressin, B-100 protein), 2-amino-5-phosphonic nivalonic acid (6- AGC) |
Long-term (lifelong) memory |
Inhibitors that irreversibly violate it are not known. Partially inhibited by atropine, diisopropyl fluorophosphate, scopolamine |
Short-term memory
Short-term memory, which analyzes information coming from various sensory organs, and its processing, is realized with the participation of synaptic contacts. This seems obvious, since the time during which these processes occur is incommensurable with the time of synthesis of new macromolecules. This is confirmed by the ability to inhibit short-term memory by synaptic inhibitors, and its insensitivity to protein and RNA synthesis inhibitors.
The process of consolidation takes a longer time and does not fit into a strictly defined interval (lasting from several minutes to several days). Probably, the duration of this period is influenced both by the quality of information and the state of the brain. Information that the brain deems inessential does not undergo consolidation and disappears from memory. It remains a mystery how the question of the value of information is decided and what are the real neurochemical mechanisms of the consolidation process. The very duration of the consolidation process allows us to consider that it is a constant state of the brain that continuously carries out the "thought process". The diverse nature of the information entering the brain for analysis, and the wide range of different inhibitory mechanisms of the consolidation process, suggest that at this stage a variety of neurochemical mechanisms are involved in the interaction.
The use of compounds indicated in the table as inhibitors of the consolidation process causes amnesia (loss of memory) in the experimental animals - the inability to reproduce the developed behavioral skill or to present the information obtained for use.
Interestingly, some inhibitors manifest themselves after the presentation of memorized information (retrograde amnesia), and others - when applied in the preceding period (anterograde amnesia). Widely known experiments on teaching chickens to distinguish grain from inedible, but similar in size objects. Introduction to the brain of chicks inhibitor protein synthesis of cycloheximide did not interfere with the learning process, but completely prevented the fixation of the skill. In contrast, the administration of inhibitors of Na pump (Na / K-ATPase) of ouabain completely inhibited the learning process without influencing the already formed skills. This means that the N-pump is involved in the formation of short-term memory, but does not participate in the consolidation processes. Moreover, the results of experiments with cycloheximide indicate that the synthesis of new protein molecules is necessary for the implementation of consolidation processes, but is not needed for the formation of short-term memory.
Consequently, training during the formation of short-term memory includes the activation of certain neurons, and consolidation - the creation of long-term interneuronal networks, to consolidate the interaction in which the synthesis of special proteins is necessary. It is not to be expected that these proteins will be carriers of specific information, their formation may be "just" an incentive for the activation of inter-neural connections. How consolidation leads to the formation of a long-term memory that can not be disturbed, but can be reproduced on demand, remains unclear.
At the same time, it is clear that the creation of a strong skill is the ability of the neuron population to form a network in which signal transmission becomes most likely, and this ability of the brain can persist for a long time. The presence of one such interneuronal network does not prevent Neurons from getting involved in similar other networks. Therefore, it is clear that the analytical capabilities of the brain are very large, if not unlimited. It is also clear that the realization of these abilities depends on the intensity of training, especially during the maturation of the brain in ontogeny. With age, the ability to learn falls.
Learning is closely related to the ability to plasticity - the ability of synaptic contacts to functional changes occurring in the process of functioning, aimed at synchronization of neuronal activity and the creation of inter-neural networks. The manifestation of plasticity is accompanied by the synthesis of specific proteins that perform known (eg, receptor) or unknown functions. One of the participants in the implementation of this program is the S-100 protein, which belongs to annexins and is found in the brain in especially large quantities (it derives its name from the ability to remain soluble at 100% saturation with ammonium sulfate at neutral pH values). Its content in the brain is several orders of magnitude greater than in other tissues. It accumulates mainly in glial cells and is found near synaptic contacts. The protein content of S-100 in the brain begins to increase 1 hour after training and reaches a maximum in 3-6 hours, remaining at a high level for several days. The introduction of antibodies to this protein in the ventricles of rat brain disrupts the learning ability of animals. All this allows us to consider the protein S-100 as a participant in the creation of inter-neural networks.
Molecular mechanisms of plasticity of the nervous system
The plasticity of the nervous system is defined as the ability of neurons to perceive signals from the external environment that alter the hard determinism of the genome. Plasticity implies the possibility of changing the functional program for the interaction of neurons in response to changes in the external environment.
Molecular mechanisms of plasticity are manifold. Let's consider the main ones on the example of the glutamatergic system. At the glutamatergic synapse, various receptors, both ionotropic and metabotropic, are simultaneously found. The release of glutamate into the synaptic cleft during excitation leads to the activation of kainate and AMPA-activated ionotropic receptors that cause depolarization of the postsynaptic membrane. At the magnitude of the transmembrane potential corresponding to the resting potential, NMDA receptors are not activated by glutamate because their ion channels are blocked. For this reason, NMDA receptors do not have a chance for immediate activation. However, when the synaptic membrane begins to depolarize, magnesium ions are removed from the binding site, which sharply increases the affinity of the receptor for glutamate.
Activation of NMDAA receptors causes the entry of calcium into the postsynaptic zone through an ion channel belonging to the NMDA receptor molecule. The calcium input is also observed through the potential-dependent Ca channels, which are activated due to the work of kainate and AMPA glutamate receptors. As a result of the aggregate of these processes in the post-synaptic zone, the content of calcium ions increases. This signal is too weak to alter the activity of multiple enzymes that are sensitive to calcium ions, but it is significant enough to activate the phospholipase C, the substrate of which is phosphoinositol, and cause the accumulation of inositol phosphates and the activation of inositol-3-phosphate-dependent calcium release from the endoplasmic reticulum.
Thus, the activation of ionotropic receptors not only causes depolarization of the membrane in the postsynaptic zone, but also creates conditions for a significant increase in the concentration of ionized calcium. In the meantime, glutamate activates in the synaptic region and metabotropic receptors. As a result, it becomes possible to activate the corresponding G-proteins "attached" to different effector systems. Kinases, phosphorylating various targets, including ionotropic receptors, can be activated, which modifies the activity of the channel structures of these formations.
Moreover, glutamate receptors are also located on the presynaptic membrane, which also have a chance to interact with glutamate. Metabotropic receptors of this synapse region are associated with the activation of the glutamate removal system from the synaptic cleft operating on the principle of glutamate reuptake. This process depends on the activity of the N-pump, since it is a secondary active transport.
Activation of the NMDA-receptors present on the presynaptic membrane also causes an increase in the level of ionized calcium in the presynaptic region of the synaptic termination. The accumulation of calcium ions synchronizes the fusion of synaptic vesicles with the membrane, accelerating the release of the mediator into the synaptic cleft.
When a series of excitation pulses arrive at the synapse and the total concentration of free calcium ions is steadily increased, activation of Ca-dependent proteinase kalpain, which cleaves one of the structural proteins fodrin, masking glutamate receptors and preventing their interaction with glutamate, can occur. Thus, the release of the mediator into the synaptic cleft during excitation provides a variety of possibilities, the realization of which can lead to amplification or inhibition of the signal, or to its culling: the synapse operates according to a multivariant principle, and the path realized at each moment depends on a variety of different factors.
Among these possibilities is the self-tuning of the synapse for the best signal transmission, which turned out to be amplified. This process is called long-term potentiation (LTP). It consists in the fact that, with prolonged high-frequency stimulation, the responses of the nerve cell to incoming impulses prove to be strengthened. This phenomenon is one of the sides of plasticity, which is based on the molecular memory of the neuronal cell. The period of long-term potentiation is accompanied by increased phosphorylation of certain neuronal proteins by specific protein kinases. One of the results of increasing the level of calcium ions in the cell is the activation of Ca-dependent enzymes (calpain, phospholipases, Ca-calmodulin-dependent protein kinases). Some of these enzymes are related to the formation of active forms of oxygen and nitrogen (NADPH oxidase, NO synthase, etc.). As a result, an accumulation of free radicals can be registered in the activated neuron, which are regarded as secondary mediators of metabolic regulation.
An important, but not the only, result of the accumulation of free radicals in a neuronal cell is the activation of the so-called early response genes. This process is the earliest and fastest transient response of the cell nucleus to the signal of free radicals, the activation of these genes occurs in 5-10 minutes and lasts several hours. These genes include the groups c-fos, c-jun, c-junB, zif / 268, etc. They encode several extensive families of specific transcriptional proteins.
Activation of immediate response genes occurs with the participation of the nuclear factor NF-kV, which must penetrate into the nucleus through the nuclear membrane for the realization of its action. Its penetration is hampered by the fact that this factor, which is a dimer of two proteins (p50 and p65), is in the complex with the protein inhibitor in the cytoplasm and is unable to penetrate into the nucleus. The inhibitory protein is a substrate for phosphorylation by a specific protein kinase, and then dissociates from the complex, which opens the way for the NF-KB B nucleus. The activating co-factor of protein kinase is hydrogen peroxide, so the free radical wave, capturing the cell, causes a number of the processes described above, leading to the activation of early response genes. Activation of c-fos can also cause the synthesis of neurotrophins and the formation of neurites and new synapses. Long-term potentiation caused by high-frequency stimulation of the hippocampus leads to activation of zif / 268, encoding the Zn-sensitive DNA-binding protein. NMDA receptor antagonists block the long-term potentiation and actives of zif / 268.
One of the first who undertook in 1949 an attempt to understand the mechanism of analyzing information in the brain and develop a strategy of behavior was SO Hebb. He suggested that in order to perform these tasks, the functional association of neurons - the local interneuronal network - should be formed in the brain. Refined and deepened these representations M. Rozenblat (1961), who formulated the hypothesis "Unsupervised correlation base learning". According to the ideas developed by him, in the case of generation of a series of discharges, neurons can be synchronized by the association of certain (often morphologically distant from each other) cells by self-tuning.
Modern neurochemistry confirms the possibility of such self-tuning of neurons at a common frequency, explaining the functional significance of the series of exciting "discharges" for creating inter-neural circuits. Using an analogue of glutamate with a fluorescent label and armed with modern technology, it was possible to show that even with the stimulation of one synapse, excitation can also spread to sufficiently remote synaptic structures due to the formation of the so-called glutamate wave. The condition for the formation of such a wave is the frequency of the signals in a certain frequency regime. Inhibition of the glutamate transporter increases the involvement of neurons in the synchronization process.
In addition to the glutamatergic system, which is directly related to the learning (memorization) processes, other brain systems also participate in the formation of memory. It is known that the ability to learn reveals a positive correlation with the activity of choline acetyl transferase and a negative one with an enzyme that hydrolyses this mediator with acetylcholinesterase. Choline acetyltransferase inhibitors disrupt the learning process, and cholinesterase inhibitors contribute to the development of defensive reflexes.
In the formation of memory, biogenic amines, norepinephrine and serotonin, also participate. In the development of conditioned reflexes with negative (electobolic) reinforcement, the noradrenergic system is activated, and with positive (nutritional) reinforcement, the rate of noradrenaline metabolism decreases. Serotonin, by contrast, facilitates the development of skills in conditions of positive reinforcement and adversely affects the formation of a defensive reaction. Thus, in the process of memory consolidation, serotonergic and noradrenalinergic systems are some kind of antagonists, and disturbances caused by excessive accumulation of serotonin can apparently be compensated by activation of the noradrenergic system.
The involvement of dopamine in the regulation of memory processes is multifactorial in nature. On the one hand, it has been revealed that it can stimulate the development of conditioned reflexes with negative reinforcement. On the other hand, it reduces the phosphorylation of neuronal proteins (eg, B-50 protein) and induces phosphoinositol exchange. It can be assumed that the dopaminergic system participates in memory consolidation.
Neuropeptides released in the synapse during excitation are also involved in memory formation processes. The vasoactive intestinal peptide increases the affinity of the cholinergic receptors to the mediator several thousand fold, contributing to the functioning of the cholinergic system. The vasopressin hormone released from the posterior lobe of the pituitary gland, synthesized in the supraoptic nuclei of the hypothalamus, is transferred to the posterior lobe of the pituitary gland, where it is stored in synaptic vesicles, and is then released into the blood. This hormone, as well as pituitary adrenocorticotropic hormone (ACTH), constantly function in the brain as regulators of memory processes. It should be emphasized that this effect differs from their hormonal activity - fragments of these compounds that lack this activity have the same effect on the learning process as whole molecules.
Nonpeptidic memory stimulators are almost unknown. The exception is orotate and is widely used in the clinic piracetam. The latter is a chemical analogue of gamma-aminobutyric acid and belongs to the group of so-called nootropic drugs, one of the effects of which is the enhancement of cerebral circulation.
With the study of the role of orotate in the mechanisms of memory fixation, intrigue is associated with the minds of neurochemists in the second half of the 20th century. The story began with the experiments of J. McConnell on the elaboration of planar illogical reflexes for light in primitive flatworms. After creating a stable reflex, he cut the planarius across into two parts and checked the learning ability of the same reflex in animals that regenerated from both halves. Surprise was that not only the individuals obtained from the head part had increased learning ability, but those that were regenerated from the tail were trained much faster than the control individuals. To train both, three times less time was required than for individuals regenerated from control animals. McConnell concluded that the acquired reaction is encoded by a substance that accumulates in both the head and tail portions of the planar body.
Reproduction of McConnell's results at other sites encountered a number of difficulties, as a result of which the scientist was declared a charlatan, and his articles ceased to be accepted for publication in all scientific journals. The enraged author founded his own magazine, where he published not only the results of subsequent experiments, but also cartoons on his reviewers and lengthy descriptions of the experiments he conducted in response to critical remarks. Thanks to McConnell's certainty in his rightness, modern science can return to an analysis of these original scientific data.
Attention is drawn to the fact that the tissues of the "trained" planaria exhibit an increased content of orotic acid, which is a metabolite necessary for the synthesis of RNA. The results obtained by McConnell can be interpreted as follows: the conditions for faster training create an increased content of orotate in "Trained" planarians. When investigating the learning of the regenerated planarians, they are faced not with the transfer of memory, but with the transfer of skill to its formation.
On the other hand, it turned out that when the regeneration of planarians is carried out in the presence of RNase, only the individuals obtained from the head fragment show increased learning ability. Independent experiments conducted at the end of the XX century. G. Ungar, allowed to isolate from the brain of animals with a darkness avoidance reflex, a 15-member peptide, called scotofobin (inducer of fear of darkness). Apparently, both RNA and some specific proteins are able to create conditions for triggering functional connections (interneuronal networks), similar to those that were activated in the original individual.
In 2005, McConnel's 80th birthday was celebrated, the experiments of which initiated the study of molecular memory carriers. At the turn of the 20th and 21st centuries. New methods of genomics and proteomics have appeared, the use of which has made it possible to reveal the involvement of low-molecular fragments of transport RNA in the consolidation processes.
The new facts make it possible to revise the concept of DNA non-participation in the mechanisms of long-term memory. The detection of RNA-dependent DNA polymerase in the brain tissue and the presence of a positive correlation of its activity with learning ability indicate the possibility of DNA participation in the processes of memory formation. It was found that the development of food conditioned reflexes sharply activates certain regions (genes responsible for the synthesis of specific proteins) of DNA in the neocortex. It is noted that the activation of DNA mainly affects areas rarely replicated in the genome, and is observed not only in nuclear but also in mitochondrial DNA, and in the latter - to a greater extent. The factors suppressing memory, simultaneously suppress these synthetic processes.
Some stimulants of memorization (on: Ashmarin, Stukalov, 1996)
Specificity of |
Stimulants |
|
|
Examples of substances |
|
Relatively specific agents |
Regulatory |
Vasopressin and its analogues, dipeptide pEAO, ACTH and its analogues |
Nonpeptidic |
Pyracetam, gangliosides |
|
Regulators of RNA metabolism |
Orotate, low molecular weight RNA |
|
Broad-spectrum agents |
Neuro-stimulants |
Phenylalkylamines (phenamine), |
Antidepressants |
2- (4-methyl-1-piperazinyl) -10-methyl-3,4-diazaphenoxazine dihydrochloride (azaphene) |
|
Modulators of the |
Cholinomimetics, acetylcholinesterase inhibitors |
The table shows examples of compounds that stimulate memorization.
It is possible that the study of the participation of DNA in the processes of memory formation will give a reasonable answer to the question of whether there are conditions under which the generated skills or the impressions that have arisen can be inherited. It is possible that the genetic memory of the long-standing events experienced by the ancestors lies at the base of some unexplained phenomena of the psyche.
According to a witty, though unproven view, the dream flights accompanying the final formation of the mature brain, experienced by each of us in our youth, reflect the sensation of the flight experienced by our distant ancestors at the time when they slept on trees. It's not for nothing that flying in a dream never ends with a fall - after all those distant ancestors who, when falling, did not have time to grab hold of the branches, although they experienced this sensation before death, but did not give offspring ...