Medical expert of the article
New publications
Synthesis, secretion and metabolism of catecholamines
Last reviewed: 19.10.2021
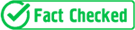
All iLive content is medically reviewed or fact checked to ensure as much factual accuracy as possible.
We have strict sourcing guidelines and only link to reputable media sites, academic research institutions and, whenever possible, medically peer reviewed studies. Note that the numbers in parentheses ([1], [2], etc.) are clickable links to these studies.
If you feel that any of our content is inaccurate, out-of-date, or otherwise questionable, please select it and press Ctrl + Enter.
The cerebral layer of the adrenal glands produces a compound far from the steroids of the structure. They contain a 3,4-dihydroxyphenyl (catechol) nucleus and are called catecholamines. These include adrenaline, norepinephrine and dopamine beta-oxytiramine.
The sequence of synthesis of catecholamines is quite simple: tyrosine → dioxyphenylalanine (DOPA) → dopamine → noradrenaline → adrenaline. Tyrosine enters the body with food, but it can also be formed from phenylalanine in the liver under the action of phenylalanine hydroxylase. The final products of the transformation of tyrosine in tissues are different. In the adrenal medulla, the process proceeds to the stage of adrenaline formation, in the endings of sympathetic nerves - norepinephrine, in some neurons of the central nervous system the synthesis of catecholamines is completed by the formation of dopamine.
The conversion of tyrosine to DOPA is catalyzed by tyrosine hydroxylase, cofactors of which are tetrahydro-biopterin and oxygen. It is believed that it is this enzyme that limits the speed of the entire process of catecholamine biosynthesis and is inhibited by the end products of the process. Tyrosine hydroxylase is the main object of regulatory effects on the biosynthesis of catecholamines.
The conversion of DOPA to dopamine is catalyzed by the enzyme DOPA-decarboxylase (cofactor-pyridoxal phosphate), which is relatively non-specific and decarboxylates other aromatic L-amino acids. However, there are indications of the possibility of modifying the synthesis of catecholamines by changing the activity and this enzyme. In some neurons there are no enzymes for the further conversion of dopamine, and it is the final product. Other tissues contain dopamine-beta-hydroxylase (cofactors are copper, ascorbic acid and oxygen), which converts dopamine into noradrenaline. In the adrenal medulla (but not at the end of the sympathetic nerves) there is phenylethanolamine - methyltransferase, which generates adrenaline from norepinephrine. The donor of the methyl groups in this case is S-adenosylmethionine.
It is important to remember that the synthesis of phenylethanolamine-N-methyltransferase is induced by glucocorticoids that enter the cerebral layer from the cortical layer through the portal venous system. In this, perhaps, lies the explanation of the fact of combining two different endocrine glands in one organ. The value of glucocorticoids for adrenaline synthesis is emphasized by the fact that adrenal medulla cells producing noradrenaline are located around arterial vessels, whereas adrenaline-producing cells receive blood mainly from venous sinuses located in the cortical layer of the adrenals.
The disintegration of catecholamines proceeds mainly under the influence of two enzyme systems: catechol-O-methyltransferase (COMT) and monoamine oxidase (MAO). The main ways of the decay of epinephrine and norepinephrine are shown schematically in Fig. 54. Under the action of COMT in the presence of a donor of methyl groups of S-adrenosylmethionine, catecholamines are converted into normetanephrine and metanephrine (3-O-methyl derivatives of norepinephrine and epinephrine), which under the influence of MAO pass into aldehydes and then (in the presence of aldehyde oxidase) to vanilla-almond acid (IUD) - the main product of the disintegration of norepinephrine and epinephrine. In the same case, when catecholamines are initially exposed to MAO, rather than COMT, they are converted to 3,4-dioximindale aldehyde, and then under the influence of aldehyde oxidase and COMT - 3,4-dihydroximidic acid and IUD. In the presence of alcohol dehydrogenase from catecholamines, 3-methoxy-4-hydroxyphenylglycol, which is the main end product of adrenaline and norepinephrine degradation in the central nervous system, can be formed.
The decomposition of dopamine proceeds similarly, except that its metabolites are devoid of the hydroxyl group of the beta-carbon atom, and therefore homovaniline (HVA) or 3-methoxy-4-hydroxyphenylacetic acid is formed instead of vanilla-mandelic acid.
The existence of a quinoid pathway for the oxidation of the molecule of catecholamines, on which intermediate products with a pronounced biological activity, can also be postulated.
The norepinephrine and epinephrine formed under the action of cytosolic enzymes in the endings of the sympathetic nerves and the adrenal medulla enter the secretory granules, which protects them from the action of degradation enzymes. The capture of catecholamines with granules requires energy costs. In chromaffin granules of the adrenal medulla, catecholamines are strongly bound to ATP (4: 1 ratio) and specific proteins - chromogranins, which prevents the diffusion of hormones from the granules into the cytoplasm.
A direct stimulus to the secretion of catecholamines is, apparently, the penetration of calcium into the cell, stimulating exocytosis (fusion of the granule membrane with the cell surface and their rupture with the complete release of soluble content - catecholamines, dopamine-beta-hydroxylase, ATP and chromogranins - into the extracellular fluid) .
Physiological effects of catecholamines and the mechanism of their action
The effects of catecholamines begin with interaction with specific receptors of target cells. If the receptors of thyroid and steroid hormones are localized inside the cells, the catecholamine receptors (as well as acetylcholine and peptide hormones) are present on the outer cell surface.
It has long been established that for some reactions, adrenaline or noradrenaline is more effective than synthetic catecholamine isoproterenol, whereas for others, the effect of isoproterenol is superior to that of epinephrine or norepinephrine. On this basis, a concept was developed for the presence in the tissues of two types of adrenoreceptors: alpha and beta, and in some of them only one of these two types can be present. Isoproterenol is the most potent agonist of beta-adrenoreceptors, whereas the synthetic compound phenylephrine is the most potent agonist of alpha-adrenoreceptors. Natural catecholamines - adrenaline and norepinephrine - are able to interact with both types of receptors, however adrenaline shows a greater affinity for beta, and norepinephrine to alpha receptors.
Catecholamines activate cardiac beta-adrenoreceptors more than beta smooth muscle receptors, which made it possible to subdivide the beta-type into subtypes: beta1 receptors (heart, fat cells) and beta2 receptors (bronchi, blood vessels, etc.). The action of isoproterenol on beta1 receptors exceeds the action of adrenaline and norepinephrine by only 10 times, whereas on beta2 receptors it acts 100-1000 times stronger than natural catecholamines.
The use of specific antagonists (phentolamine and phenoxybenzamine against alpha and propranolol for beta receptors) confirmed the adequacy of the classification of adrenoreceptors. Dopamine is able to interact with both alpha- and beta-receptors, but in different tissues (brain, pituitary, vessels), there are also found dopaminergic receptors whose specific blocker is haloperidol. The number of beta receptors ranges from 1000 to 2000 per cell. Biological effects of catecholamines mediated by beta receptors are associated, as a rule, with the activation of adenylate cyclase and an increase in the intracellular content of cAMP. The receptor and enzyme, although they are connected functionally, but represent different macromolecules. In the modulation of adenylate cyclase activity, under the influence of the hormone-receptor complex, guanosine triphosphate (GTP) and other purine nucleotides are involved. By increasing the activity of the enzyme, they seem to reduce the affinity of the beta receptors for agonists.
The phenomenon of increasing the sensitivity of denervated structures has long been known. Conversely, prolonged exposure to agonists decreases the sensitivity of target tissues. The study of beta receptors allowed to explain these phenomena. It was shown that prolonged action of isoproterenol leads to a loss of sensitivity of adenylate cyclase due to a decrease in the number of beta receptors.
The process of desensitization does not require the activation of protein synthesis and is probably due to the gradual formation of irreversible hormone-receptor complexes. On the contrary, the administration of 6-oxidofamin, which breaks sympathetic ends, is accompanied by an increase in the number of reacting beta receptors in tissues. It is not excluded that an increase in sympathetic nervous activity determines the age-related desensitization of blood vessels and fatty tissue in relation to catecholamines.
The number of adrenoreceptors in different organs can be controlled by other hormones. Thus, estradiol increases, and progesterone reduces the number of alpha-adrenergic receptors in the uterus, which is accompanied by a corresponding increase and decrease in its contractile response to catecholamines. If the intracellular "second messenger" formed by the action of β-receptor agonists is certainly cAMP, then the case of the transmitter of alpha-adrenergic influences is more complicated. It is assumed that there are various mechanisms: a decrease in the level of cAMP, an increase in the content of cAMP, a modulation of the cellular dynamics of calcium,
To reproduce a variety of effects in the body, doses of epinephrine, which are 5-10 times lower than norepinephrine, are usually required. Although the latter is more effective for a- and beta1-adrenergic receptors, it is important to remember that both endogenous catecholamines are able to interact with both the alpha and beta receptors. Therefore, the biological response of this body to adrenergic activation largely depends on the type of receptors present in it. However, this does not mean that selective activation of the nervous or humoral link of the sympathetic-adrenal system is impossible. In most cases there is an intensified activity of its various links. So, it is commonly believed that hypoglycemia activates the cerebral layer of the adrenal glands reflexively, whereas a decrease in arterial pressure (postural hypotension) is accompanied mainly by the release of norepinephrine from the endings of the sympathetic nerves.
Adrenoreceptors and the effects of their activation in various tissues
System, organ |
Adrenoceptor type |
Reaction |
The cardiovascular system: |
||
A heart |
Beta |
Increase in the frequency of contractions, conduction and contractility |
Arterioles: |
||
Skin and mucous membranes |
Alpha |
Reduction |
Of skeletal muscles |
Beta |
Extension Reduction |
Abdominal organs |
Alpha (more) |
Reduction |
Beta |
Expansion |
|
Veins |
Alpha |
Reduction |
Respiratory system: |
||
Bronchial muscles |
Beta |
Expansion |
Digestive system: |
||
Stomach |
Beta |
Decreased motor function |
Intestines |
Alpha |
Reduction of sphincters |
Spleen |
Alpha |
Reduction |
Beta |
Relaxation |
|
Externally secretive part of the pancreas |
Alpha |
Decreased secretion |
Genitourinary system: |
Alpha |
Sphincter reduction |
Bladder |
Beta |
Relaxing the exorcist muscle |
Male sexual organs |
Alpha |
Ejaculation |
Eyes |
Alpha |
Pupil dilated |
Leather |
Alpha |
Increased sweating |
Salivary glands |
Alpha |
Isolation of potassium and water |
Beta |
Secretion of amylase |
|
Endocrine glands: |
||
Islets of the pancreas |
||
Beta cells |
Alpha (more) |
Decreased insulin secretion |
Beta |
Increased insulin secretion |
|
Alpha cells |
Beta |
Increased secretion of glucagon |
8-cells |
Beta |
Increased secretion of somatostatin |
The hypothalamus and the pituitary: |
||
Somatotrophs |
Alpha |
Increased secretion of STH |
Beta |
Reduced secretion of STH |
|
Lactotrophs |
Alpha |
Decreased secretion of prolactin |
Thyrotrophs |
Alpha |
Decreased secretion of TSH |
Corticotrophs |
Alpha |
Increased secretion of ACTH |
beta | Decreased secretion of ACTH | |
Thyroid: |
||
Follicular cells |
Alpha |
Decreased secretion of thyroxine |
Beta |
Increased secretion of thyroxine |
|
Parafollicular (K) cells |
Beta |
Increased secretion of calcitonin |
The parathyroid glands |
Beta |
Increased secretion of PTH |
Kidneys |
Beta |
Increased renin secretion |
Stomach |
Beta |
Increase gastrin secretion |
BX |
Beta |
Increase in oxygen consumption |
Liver |
? |
Increase in glycogenolysis and gluconeogenesis from glucose yield; increase ketogenesis with the release of ketone bodies |
Adipose tissue |
Beta |
The increase in lipolysis with the release of free fatty acids and glycerol |
Skeletal muscles |
Beta |
Increase in glycolysis with the release of pyruvate and lactate; decrease in proteolysis with a decrease in the yield of alanine, glutamine |
It is important to consider that the results of intravenous administration of catecholamines do not always adequately reflect the effects of endogenous compounds. This applies mainly to noradrenaline, since in the body it is released mainly not into the blood, but directly into the synaptic clefts. Therefore, endogenous noradrenaline activates, for example, not only vascular alpha receptors (increased blood pressure), but also beta-receptors of the heart (rapid heartbeat), whereas the introduction of norepinephrine from the outside mainly leads to activation of vascular alpha receptors and reflex (through vagus) deceleration palpitations.
Low doses of epinephrine activate mainly the beta receptors of the muscle vessels and heart, resulting in a drop in peripheral vascular resistance and increase the minute volume of the heart. In some cases, the first effect may predominate, and after administration of adrenaline, hypotension develops. In higher doses, adrenaline also activates alpha receptors, which is accompanied by an increase in peripheral vascular resistance and against the background of an increase in the minute volume of the heart leads to an increase in blood pressure. However, its effect on vascular beta receptors is also preserved. As a result, the increase in systolic pressure exceeds the similar value of diastolic pressure (increase in pulse pressure). With the introduction of even larger doses, alpha-mimetic effects of epinephrine begin to prevail: systolic and diastolic pressure increase in parallel, both under the influence of noradrenaline.
The effect of catecholamines on metabolism is made up of their direct and indirect effects. The first are realized mainly through beta-receptors. More complex processes are associated with the liver. Although the enhancement of hepatic glycogenolysis has traditionally been considered the result of beta-receptor activation, there are also data on the involvement of alpha receptors in this. Mediated effects of catecholamines are associated with the modulation of the secretion of many other hormones, for example insulin. In the action of adrenaline on its secretion, the alpha-adrenergic component clearly predominates, since it is shown that any stress is accompanied by inhibition of insulin secretion.
The combination of direct and indirect effects of catecholamines causes hyperglycemia, associated not only with an increase in the hepatic production of glucose, but also with inhibition of its utilization by peripheral tissues. Acceleration of lipolysis causes hyperlipacidemia with increased delivery of fatty acids to the liver and intensification of production of ketone bodies. The increase in glycolysis in muscles leads to an increase in the release of lactate and pyruvate into the blood, which together with glycerol released from adipose tissue serve as precursors of hepatic gluconeogenesis.
Regulation of the secretion of catecholamines. The similarity of products and ways of responding to the sympathetic nervous system and the adrenal medulla was the basis for combining these structures into a single sympathetic-adrenal system of the organism, with the release of the nervous and hormonal link. Various afferent signals are concentrated in the hypothalamus and centers of the spinal and medulla oblongata, from which efferent premisses emerge that switch to the cellular bodies of preganglionic neurons located in the lateral horns of the spinal cord at the level of the VIII cervical II-III lumbar segments.
Preganglionic axons of these cells leave the spinal cord and form synaptic connections with neurons localized in the ganglia of the sympathetic chain, or with cells of the adrenal medulla. These preganglionic fibers are cholinergic. The first fundamental difference between sympathetic postganglionic neurons and chromaffin cells of the adrenal medulla is that the latter transmit the cholinergic signal arriving to them non-nervously-conductive (postganglionic adrenergic nerves), but by humoral way, releasing adrenergic compounds into the blood. The second difference is that the postganglionic nerves produce norepinephrine, whereas the adrenal medulla are mainly adrenaline. These two substances have a different effect on the tissue.