Medical expert of the article
New publications
MRI (magnetic resonance imaging)
Last reviewed: 23.04.2024
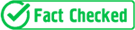
All iLive content is medically reviewed or fact checked to ensure as much factual accuracy as possible.
We have strict sourcing guidelines and only link to reputable media sites, academic research institutions and, whenever possible, medically peer reviewed studies. Note that the numbers in parentheses ([1], [2], etc.) are clickable links to these studies.
If you feel that any of our content is inaccurate, out-of-date, or otherwise questionable, please select it and press Ctrl + Enter.
MRI (magnetic resonance imaging) produces images by using a magnetic field to induce changes in proton rotation inside tissues. Usually the magnetic axes of numerous protons in tissues are randomly distributed. When they are surrounded by a strong magnetic field, as in the MRI mechanism, the magnetic axes are aligned along the field. The impact of the high-frequency pulse causes the axes of all protons to instantaneously align along the field in the high-energy state; some protons after this return back to their original state within the magnetic field. The magnitude and speed of release of energy, which takes place simultaneously with the return to the initial alignment (relaxation of T1) and with the oscillation (precession) of the protons during the process (relaxation of T2), are recorded as spatially limited by the coil (antenna) signal strengths. These tensions are used to create images. The relative intensity of the signal (brightness) of the tissues on the MP image is determined by numerous factors including the high-frequency pulse and the gradient waveforms used to produce the images characteristic of the tissue T1 and T2 characteristics and tissue proton density.
Pulse sequences are computer programs that control the high-frequency pulse and waveforms of the gradient, which determine how the image appears and how different tissues look. Images can be T1-weighted, T2-weighted or weighted by the density of the proton. For example, fat appears bright (high signal strength) on T1-weighted images and relatively dark (low signal strength) on T2 images; water and liquids appear as an intermediate signal intensity on T1-weighted images and bright on T2-weighted images. T1-weighted images optimally demonstrate the normal anatomy of the soft tissue (the fat planes are well manifested as a high signal intensity) and fat (for example, to confirm the presence of fat-containing mass). T2-weighted images optimally demonstrate fluid and pathology (eg, tumors, inflammation, trauma). In practice, T1 - and T2-weighted images provide additional information, so both are important for the characterization of pathology.
Indications for MRI (magnetic resonance imaging)
In order to advance the vascular structures (magnetic resonance angiography) and to help characterize inflammation and tumors, contrast can be used. The most frequently used agents are gadolinium derivatives, which have magnetic properties that affect the time of proton relaxation. Gadolinium agents can cause headache, nausea, pain and cold feeling at the injection site, distortion of taste sensations, dizziness, vasodilation and reduced threshold of seizures; Serious contrast reactions appear rarely and are much less common than those occurring in prion-containing contrast agents.
MRI (magnetic resonance imaging) is preferable to CT when it is important to address the contrast of soft tissue - for example, to assess intracranial abnormalities, spinal deviations or spinal cord abnormalities or to assess suspected musculoskeletal tumors, inflammation, trauma or internal joint disorder the imaging of intraarticular structures may involve the injection of a gadolinium agent into the joint). MRI also helps in assessing liver pathologies (eg, tumors) and female reproductive organs.
Contraindications to MRI (magnetic resonance imaging)
The first relative contraindication to MRI is the presence of an implanted material that can be damaged by powerful magnetic fields. These materials include ferromagnetic metal (containing iron), magnetically activated or electronically controlled medical devices (for example, pacemakers, implantable cardioverter defibrillators, cochlear implants), and wires or materials from non-ferromagnetic metals electronically controlled (for example, pacemaker wires, some pulmonary artery catheters). Ferromagnetic material can shift due to a strong magnetic field and damage the nearby organ; displacement is even more likely if the material was present there for less than 6 weeks (before the formation of scar tissue). Ferromagnetic material can also cause image distortion. Magnetically activated medical devices can malfunction. In conductive materials, magnetic fields can produce a flux that, in turn, can cause heat. The compatibility of an MRI device or object may be specific to a particular type of device, component, or manufacturer; Preliminary testing is usually required. Also, MRI mechanisms of different magnetic field strengths have a different effect on materials, so that security for one mechanism does not guarantee security for another.
Thus, a ferromagnetic object (for example, an oxygen reservoir, some IV poles) at the entrance to the scanning room can be drawn into the magnetic channel at high speed; the patient may be injured, and the separation of the object from the magnet may become impossible.
The mechanism of MRI is a tense, closed space that can cause claustrophobia even in patients who do not suffer from it. Also, some patients with high weight can not fit on the table or in the car. For the most restless patients, a preliminary sedative (eg, alprazolam or lorazepam 1-2 mg orally) would be effective 15 to 30 minutes before the scan.
If there are certain indications, several unique methods of MRI are used.
A gradient echo is a pulse sequence used for rapid imaging (for example, magnetic resonance angiography). The movement of blood and cerebrospinal fluid produces strong signals.
Repeated flat mapping is an ultra-fast technique used for diffusion, perfusion and functional mapping of the brain.