Osteoarthritis: how are the articular cartilages arranged?
Last reviewed: 23.04.2024
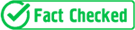
All iLive content is medically reviewed or fact checked to ensure as much factual accuracy as possible.
We have strict sourcing guidelines and only link to reputable media sites, academic research institutions and, whenever possible, medically peer reviewed studies. Note that the numbers in parentheses ([1], [2], etc.) are clickable links to these studies.
If you feel that any of our content is inaccurate, out-of-date, or otherwise questionable, please select it and press Ctrl + Enter.
Normal articular cartilage performs two main functions: the absorption of pressure by deformation during mechanical stress and ensuring the smoothness of articular surfaces, which allows you to minimize friction when moving in the joint. This is ensured by the unique structure of articular cartilage, which consists of chondro-ita immersed in the extracellular matrix (ECM).
The normal articular cartilage of an adult can be divided into several layers, or zones: a surface, or tangential, zone, a transition zone, a deep, or a radial zone and a calcified zone. The layer between the surface and transition zones and especially between the transition and deep zones has no clear boundaries. The connection between uncalcified and calcified articular cartilage is called the "wavy border" - this is the line determined by staining the decalcified tissue. The calcified zone of cartilage is a relatively constant proportion (6-8%) in the total height of the crescents. The total thickness of articular cartilage, including the zone of calcified cartilage, varies depending on the load on a certain area of the joint surface and the type of joint. Intermittent hydrostatic pressure in the subchondral bone plays an important role in maintaining the normal structure of the cartilage, slowing ossification.
Chondrocytes constitute approximately 2-3% of the total tissue mass; in the surface (tangential) zone they are located along, and in the deep (radial) zone - perpendicular to the surface of the cartilage; in the transition zone, chondrocytes form groups of 2-4 cells scattered throughout the matrix. Depending on the area of the articular cartilage, the density of the location of the chondrocytes varies - the highest cell density in the surface zone, the lowest in the calcified one. In addition, the density of the cell distribution varies from joint to joint, it is inversely proportional to the thickness of the cartilage and the load experienced by its corresponding site.
The most superficially located chondrocytes are disk-shaped and form in the tangential zone several layers of cells located below a narrow strip of matrix; Deeply located cells of this zone tend to have more uneven contours. In the transition zone, chondrocytes have a spherical shape, sometimes they are combined into small groups scattered in the matrix. Chondrocytes of the deep zone are predominantly ellipsoidal in shape, grouped into radially arranged chains of 2-6 cells. In the calcified zone they are distributed even more sparingly; some of them are necrotic, although most are viable. The cells are surrounded by an uncalcified matrix, the intercellular space is calcified.
Thus, the human articular cartilage consists of hydrated ECM and cells immersed in it, which constitute 2-3% of the total tissue volume. Since cartilaginous tissue does not have blood and lymphatic vessels, the interaction between cells, the delivery of nutrients to them, the removal of metabolic products is carried out by diffusion through the ECM. Despite the fact that the metabolic chondrocytes are very active, they do not normally divide in adult people. Chondrocytes exist in an oxygen-free environment, believe that their metabolism is carried out predominantly anaerobically.
Each chondrocyte is considered as a separate metabolic unit of cartilage, isolated from neighboring cells, but responsible for the production of VKM elements in the immediate vicinity of the given cell and maintaining its composition.
In VKM three departments are distinguished, each of which has a unique morphological structure and a certain biochemical composition. VKM, immediately adjacent to the chasmic cell of the chondrocyte, is called a pericellular, or lacunary, matrix. It is characterized by a high content of aggregates of proteoglycans associated with the cell by the interaction of hyaluronic acid with CD44-like receptors, and the relative absence of organized collagen fibrils. Directly with the pericellular matrix, a territorial, or capsular, matrix is contiguous, which consists of a network of intersecting fibrillar collagens that encapsulate individual cells or (sometimes) groups of cells, forming chondron, and probably provides special mechanical support for the cells. Contact of chondrocytes with the capsular matrix is carried out by means of numerous cytoplasmic processes rich in microfilaments, and also by specific matrix molecules such as anchorin and CD44-like receptors. The largest and most remote from the basal membrane of the chondrocyte is the ECM-interterritorial matrix containing the largest number of collagen fibrils and proteoglycans.
The division of ECM into departments is more clearly delineated in the articular cartilage of an adult than in immature articular cartilage. The relative size of each department varies not only in different joints, but even within the same cartilage. Each chondrocyte produces a matrix surrounding it. According to studies, chondrocytes of mature cartilaginous tissue carry out active metabolic control over their pericellular and territorial matrices, less actively they control the interterritorial matrix, which can be metabolically "inert."
As indicated earlier, articular cartilage mainly consists of a vast VKM, synthesized and regulated by chondrocytes. Tissue macromolecules and their concentration change during life in accordance with changing functional needs. However, it remains unclear: cells synthesize the entire matrix simultaneously or in certain phases in accordance with physiological needs. The concentration of macromolecules, the metabolic balance between them, the relationship and interaction determine the biochemical properties, and hence the function of the articular cartilage within one joint. The main component of ECM of the articular cartilage of an adult is water (65-70% of the total mass), which is firmly bound inside it due to the special physical properties of the cartilage tissue macromolecules included in collagens, proteoglycans and non-collagen glycoproteins.
Biochemical composition of cartilage
Collagen fibers consist of molecules of collagen fibrillar protein. In mammals, the proportion of collagen accounts for one-fourth of all proteins in the body. Collagen forms fibrillar elements (collagen fibrils), consisting of structural subunits, called tropocollagen. The tropocollagen molecule has three chains that form a triple helix. This structure of the tropocollagen molecule, as well as the structure of the collagen fiber, when these molecules are parallel in the longitudinal direction with a constant shift of about 1/4 of the length and provide high elasticity and strength to the tissues in which they are located. Currently, 10 genetically different types of collagen are known, differing in the chemical structure of the a-chains and / or their collection in the molecule. The most studied first four types of collagen are capable of forming up to 10 molecular isoforms.
Collagen fibrils are part of the extracellular space of most types of connective tissue, including cartilaginous tissue. Within the insoluble three-dimensional network, other more soluble components, such as proteoglycans, glycoproteins and tissue-specific proteins, are "tangled" from the collapsing collagen fibrils; sometimes they are covalently bound to collagen elements.
Collagen molecules organized in fibrils constitute about 50% of the organic dry residue of cartilage (10-20% of native cartilage). In mature cartilage, about 90% of the collagens are type II collagens, which are found only in certain tissues (eg, vitreous, embryonic spinal cord). Collagen type II refers to the first class (forming fibrils) of collagen molecules. In addition to him, in the mature articular cartilage of a person collagen IX, XI type and in a small number of VI type are also found. The relative amount of IX type collagen fibers in collagen fibrils decreases from 15% in the cartilage of the fetus to about 1% in the mature cartilage of the bull.
Molecules of collagen I type consist of three identical polypeptide a, (II) chains, synthesized and secreted in the form of precollagen precursor. Once the ready-made collagen molecules are released into the extracellular space, they form fibrils. In mature articular cartilage collagen type II form fibrillar arcades, in which more "thick" molecules are located in deep layers of tissue, and more "thin" - horizontally in the surface layers.
In the procollagen gene of type II, an exon encoding a cysteine-rich N-terminal propeptide was found. This exon is not expressed in mature cartilage, but in the early stages of development (prechondrogenesis). Due to the presence of this exon, the procollagen II type molecule (type II A) is longer than type II collagen. Probably, the expression of this type of procollagen inhibits the accumulation of elements in the ECM of the articular cartilage. It may play a role in the development of cartilage pathology (for example, inadequate reparative response, osteophyte formation, etc.).
A network of type II collagen fibrils provides a tensile strength function and is necessary to maintain the volume and shape of the tissue. This function is enhanced by covalent and cross-linking between collagen molecules. In VKM, the lysiloxidase enzyme forms an aldehyde from hydroxylizine, which is then converted to a multivalent amino acid hydroxylisyl-pyridinoline, which forms cross-links between the chains. On the one hand, the concentration of this amino acid rises with age, however, in mature cartilage it practically does not change. On the other hand, in the articular cartilage, an increase in the concentration of cross-links of different types with age is formed with age, formed without the participation of enzymes.
About 10% of the total amount of collagen cartilage tissue is the so-called minor collagens, which in many respects determine the unique function of this tissue. Collagen IX type belongs to the III class of short-spiral molecules and to a unique group of FACIT-collagens (Fibril-Associated Collagen with Interrupted Triple-helices) with fused triple helix. It consists of three genetically different chains. One of them - a 2- chain - is glycosylated simultaneously with chondroitin sulfate, which makes this molecule simultaneously proteoglycan. Between segments of the collagen type IX spiral and type II collagen, both mature and immature hydroxypyridine cross-links are detected. Collagen IX can also function as an intermolecular-interfibrillar "connector" (or bridge) between adjacent collagen fibrils. Collagen IX molecules form cross-links between themselves, which increases the mechanical stability of the fibrillar three-dimensional network and protects it from the effects of enzymes. They also provide resistance to deformation, limiting the swelling of proteoglycans within the network. In addition to the anionic CS chain, the collagen IX molecule contains a cationic domain that provides a high-charge fibril and a tendency to interact with other matrix macromolecules.
Collagen XI type is only 2-3% of the total mass of collagens. It belongs to the first class (forming fibrils) of collagens and consists of three different a-chains. Together with collagen types II and IX, type X collagen forms heterotic fibrils of articular cartilage. Molecules of collagen XI type are found inside collagen fibrils of type II with the help of immunoelectromicroscopy. Perhaps they organize collagen type II molecules, controlling the lateral growth of fibrils and determining the diameter of the heterotypic collagen fibril. In addition, collagen XI is involved in the formation of cross-links, but even in mature cartilage, the transverse bonds remain in the form of immature divalent ketoamines.
A small amount of type VI collagen, another representative of class III of short-span molecules, was found in the articular cartilage. Collagen type VI forms various microfibrils and, possibly, is concentrated in the capsular matrix of the chondron.
Proteoglycans are proteins to which at least one glycosaminoglycan chain is covalently attached. Proteoglycans belong to one of the most complex biological macromolecules. The most extensive proteoglycans are present in the cartilage VKM. "Entangled" inside the network of collagen fibrils, hydrophilic proteoglycans fulfill their main function - they inform the cartilage of the ability to reversibly deform. It is believed that proteoglycans carry out a number of other functions, the essence of which is not completely clear.
Aggrecan is the main proteoglycan of articular cartilage: it is about 90% of the total mass of proteoglycans in the tissue. Its core protein of 230 kD is glycosylated by a number of covalently linked glycosaminoglycan chains, as well as N-terminal and C-terminal oligosaccharides.
Glycosaminoglycan chains of articular cartilage, which constitute about 90% of the total mass of macromolecules, is keratan sulfate (a sequence of sulfated disaccharide N-acetylglucosaminogalactose with multiple sulphated sites and other monosaccharide residues such as sialic acid) and chondroitin sulfate (is a sequence of N-acetylgalactosamine-glucuronic acid disaccharide with sulfate ester attached to each fourth or sixth carbon atom of N-acetyl lactosamine).
The core of the aggregate contains three globular (G1, G2, G3) h two interglobular (E1 and E2) domains. The N-terminal region contains G, and G2 domains separated by an E1 segment of 21 nm in length. C3-domain located at the C-terminus, separated from G 2 longer (about 260 nm) E2 segment that bears more than 100 chondroitin sulfate chains of about 15-25 keratan sulfate chains and O-linked oligosaccharides. N-linked oligosaccharides found mainly within G1- and C2 domains and E1-segment, as well as near the G 3 -regiona. Glycosaminoglycans are grouped in two regions: the longest (the so-called region, rich in chondroitin sulfates) contains chains of chondroitin sulfates and about 50% of keratan sulfate chains. The region rich in keratan sulfates is localized on the E 2 segment near the G1 domain and precedes the region rich in chondroitin sulfates. Aggregan molecules also contain phosphate esters, localized primarily on xylose residues, which attach chondroitin sulfate chains to the core protein; they are also found on the serine residues of the core protein.
The C-terminal segment of the C3-domain is highly homologous to the lectin, so that the proteoglycan molecules can be fixed in the ECM by binding to certain hydrocarbon structures.
In recent studies, an exon coding for the EGF-like (epidermal growth factor) subdomain within G 3 was observed . Using anti-EGF polyclonal antibodies, an EGF-like epitope was localized within a peptide of 68 kD in the aggregate of the human articular cartilage. However, its functions require clarification. This subdomain is also found in the structure of adhesion molecules controlling the migration of lymphocytes. Only about one third of the aggrecan molecules isolated from mature human articular cartilage contain intact C 3 domains of; probably this is due to the fact that in the ECM, the aggrecan molecules can be reduced in size by the enzyme route. The further fate and function of the split fragments are unknown.
The main functional segment is an aggrecan molecule glikozaminoglikannesuschy E 2 -segment. The site, rich in keratan sulfates, contains the amino acids proline, serine and threonine. Most serine and threonine residues are O-glycosylated with N-acetylgalactosamine residues, they trigger the synthesis of some oligosaccharides that are embedded in the keratan sulfate chains, thereby prolonging them. The rest of the E 2 segment contains more than 100 serine-glycine sequences in which the series provides attachment to the xylosyl residues at the start of the chondroitin sulfate chains. Usually both chondroitin-6-sulphate and chondroitin-4-sulfate exist simultaneously within the same molecule of proteoglycan, from the ratio varies depending on the localization of the cartilaginous tissue and the human age.
The structure of the aggrecan molecules in the matrix of the articular cartilage of a person undergoes a number of changes in the process of maturation and aging. The aging-related changes include a decrease in the hydrodynamic size as a result of changes in the average chain length of chondroitin sulfates, an increase in the number and length of keratan sulfate chains. A number of changes in the aggrecan molecule also undergo the action of proteolytic enzymes (eg, aggrecanase and stromelysin) on the core protein. This leads to a progressive decrease in the average length of the core protein of the aggrecan molecules.
Aggrecan molecules are synthesized by chondrocytes and secreted in the ECM, where they form aggregates stabilized by molecules of binding proteins. This aggregation includes highly specific non-covalent and cooperative interactions between the glucuronic acid filament and nearly 200 molecules of aggrecans and binding proteins. Glucuronic acid is an extracellular, non-sulphonated linear glycosaminoglycan with a large molecular weight, consisting of a number of sequentially bound molecules of N-acetylglucamine and glucuronic acid. The coupled loops of the G1 domain of aggrecan interact reversibly with five consecutively arranged hyaluronic acid disaccharides. The binding protein, which contains similar (high-homologous) paired loops, interacts with the C1 domain and the hyaluronic acid molecule and stabilizes the structure of the aggregate. The C1-domain-hyaluronic acid-binding protein complex forms a highly stable interaction that protects the G1 domain and the binding protein from the action of proteolytic enzymes. Two molecules of a binding protein with a molecular weight of 40-50 kD were identified; they differ from each other in the degree of glycosylation. Only one molecule of the binding protein is present at the hyaluronic acid-aggrecan bonding site. The third, smaller, molecule of the binding protein is formed from larger proteins by proteolytic cleavage.
About 200 molecules of aggrecan can bind to one molecule of hyaluronic acid to form an aggregate of 8 μm in length. In the cell-associated matrix, consisting of the pericellular and territorial divisions, the aggregates retain their connection with the cells by binding (through the filament of hyaluronic acid) to the CD44-like receptors on the cell membrane.
The formation of aggregates in the ECM is a complex process. The newly synthesized aggrecan molecules do not immediately manifest the ability to bind to hyaluronic acid. This can serve as a regulatory mechanism allowing newly synthesized molecules to reach the interterritorial zone of the matrix before being immobilized into large aggregates. The number of newly synthesized aggrecan molecules and binding proteins capable of forming aggregates by interacting with hyaluronic acid decreases significantly with age. In addition, with age, the size of aggregates isolated from the articular cartilage of a person is significantly reduced. This is partly due to the decrease in the average length of molecules of hyaluronic acid and aggrecan molecules.
There are two types of aggregates in the articular cartilage. The average size of aggregates of the first type is 60 S, the aggregates of the second type (rapidly precipitating "superaggregates") are 120 S. The latter is characterized by an abundance of molecules of the binding protein. The presence of these superagregates may play a big role in the functioning of the tissue; during the restoration of tissue after immobilization of the limb in the middle layers of the articular cartilage, their higher concentrations are found, in the joint affected by osteoarthritis, in the early stages of the disease their dimensions are significantly reduced.
In addition to aggrecan, articular cartilage contains a number of smaller proteoglycans. Biglikan and decorin, molecules bearing dermatan sulfates have a molecular mass of about 100 and 70 kD, respectively; the mass of their core protein is about 30 kD.
In the articular cartilage of a human, the biglucan molecule contains two chains of dermatan sulfate, while the more common decorin is only one. These molecules constitute only a small fraction of proteoglycans in the articular cartilage, although there may be as many of them as large aggregated proteoglycans. Small proteoglycans interact with other macromolecules in the ECM, including collagen fibrils, fibronectin, growth factors, etc. Decorin initially localizes on the surface of collagen fibrils and inhibits collagen fibrillogenesis. The core protein is firmly retained with the cell-binding domain of fibronectin, thereby, probably, preventing the binding of the latter to cell surface receptors (integrins). Due to the fact that both decorin and biglikan bind to fibronectin and inhibit the adhesion and migration of cells, as well as the formation of thrombi, they are able to inhibit the processes of tissue repair.
Fibromodulin of the articular cartilage is a proteoglycan with a molecular mass of 50-65 kD, associated with collagen fibrils. Its core protein, homologous to the core proteins of the decor and bigakana, contains a large amount of tyrosine sulfate residues. This glycosylated form of fibromodulin (formerly called a 59 kD matrix protein) can participate in the regulation of formation and maintenance of the structure of collagen fibrils. Fibromodulin and decorin are located on the surface of collagen fibrils. Thus, as indicated earlier, the increase in fibril diameter should be preceded by the selective removal of these proteoglycans (as well as collagen type IX molecules).
Articular cartilage contains a number of proteins in the VKM, which do not belong to either proteoglycans or collagens. They interact with other macromolecules to form a network in which most VKM molecules are incorporated.
Anchorin, a protein with a mass of 34 kD, is localized on the surface of chondrocytes and in the cell membrane, mediates the interaction between the cell and the matrix. Due to its high affinity for collagen type II, it can act as a mechanoreceptor, which transmits a signal about the changed pressure on the fibril of the chondrocyte.
Fibronectin is a component of most cartilaginous tissues, slightly different from fibronectin of the blood plasma. It is suggested that fibronectin promotes the integration of the matrix by interacting with cell membranes and other matrix constituents such as collagen type II and thrombospondin. Fragments of fibronectin negatively affect the metabolism of chondrocytes - inhibit the synthesis of aggrecan, stimulate catabolic processes. In the joint fluid of patients with osteoarthrosis, a high concentration of fibronectin fragments was found, so they can participate in the pathogenesis of the disease in later stages. Probably, fragments of other matrix molecules that bind to chondrocyte receptors also have the same effects.
The cartilage oligomeric matrix protein (OMPC), a member of the thrombospondin superfamily, is a pentamer with five identical subunits with a molecular weight of about 83 kD. They are found in large numbers in the articular cartilage, especially in the layer of proliferating cells in the growing tissue. Therefore, perhaps, OMPCH takes part in the regulation of cell growth. At a much lower concentration, they are found in the ECM of mature articular cartilage. Matrix proteins are also referred to as:
- the basic matrix protein (36 kD), which has a high affinity for chondrocytes, can mediate the interaction of cells in the ECM, for example, during tissue remodeling;
- GP-39 (39 kD) is expressed in the surface layer of articular cartilage and in the synovial membrane (its functions are unknown);
- 21 kD protein is synthesized by hypertrophied chondrocytes, interacts with X-type collagen, can function in the "wave-line" zone.
In addition, it is evident that the chondrocytes express the non-glycosylated forms of small non-aggregated proteoglycans at certain stages of cartilage development and in pathological conditions, but their specific function is currently being studied.
[10], [11], [12], [13], [14], [15]
Functional properties of cartilage of the joint
Molecules of aggrecan give articular cartilage the ability to undergo reversible deformation. They demonstrate specific interactions within the extracellular space and undoubtedly play an important role in the organization, structure and function of ECM. In the cartilaginous tissue aggrecane molecules reach a concentration of 100 mg / ml. In the cartilage, the Aggregan molecules are compressed to 20% of the volume they occupy in the solution. A three-dimensional network formed by collagen fibrils informs the tissue of its characteristic shape and prevents the increase in the volume of proteoglycans. Inside the collagen network, immobile proteoglycans carry a large negative electric charge (contain a large number of anionic groups), which allows interacting with mobile cationic groups of the interstitial fluid. Interacting with water, proteoglycans provide the so-called swelling pressure, which is counteracted by the collagen network.
The presence of water in the ECM is very important. Water determines the volume of tissue; associated with proteoglycans, it provides resistance to compression. In addition, water provides transport of molecules and diffusion in the ECM. The high density of negative charge on large proteoglycans fixed in tissue creates an "excluded volume effect". The pore size of the intra-concentrated solution of proteoglycans is so small that the diffusion of large globular proteins into the tissue is severely restricted. VKM repels small negatively charged (eg, chloride ions) and large (such as albumin and immunoglobulins) proteins. The size of cells within a dense network of collagen fibrils and proteoglycans is commensurable only with the dimensions of some inorganic molecules (for example, sodium and potassium, but not calcium).
In VKM some amount of water is present in the collagen fibrils. Physicochemical and biomechanical properties of cartilage determine extrafibrillar space. The water content in the fibrillar space depends on the concentration of proteoglycans in the extrafibrillar space and increases with a decrease in the concentration of the latter.
The fixed negative charge on proteoglycans determines the ion composition of the extracellular medium containing free cations in high concentration and free anions in low concentration. Since the concentration of the aggrecan molecules rises from the surface to the deep zone of the cartilage, the ionic environment of the tissue changes. The concentration of inorganic ions in the ECM produces a high osmotic pressure.
The properties of cartilage as a material depend on the interaction of collagen fibrils, proteoglycans and the liquid phase of the tissue. Structural and compositional changes associated with a mismatch between the processes of synthesis and catabolism, the degradation of macromolecules and physical trauma, significantly affect the material properties of cartilage and alter its function. Since the concentration, distribution and macro-molecular organization of collagens and proteoglycans vary with the depth of the cartilage zone, the biomechanical properties of each zone vary. For example, the surface area with its high collagen concentration, tangentially located fibrils, a relatively low concentration of proteoglycans has the most pronounced properties to counteract stretching, distributing the load evenly over the entire surface of the tissue. In the transitional and deep zones, a high concentration of proteoglycans imparts a tissue property to the transferring of the compression load. At the level of the "wavy line", the material properties of the cartilage vary sharply from the ductile noncalcified zone to the more rigid mineralized cartilage. In the area of the "wavy line" the strength of the tissue is provided by the collagen network. Cartilaginous fibrils do not cross the cartilaginous parts; in the area of bone-cartilage, the strength of the tissue is provided by special contours of the boundary between the zones of uncalcified and calcified cartilage in the form of irregular fingerlike outgrowths that "closes" the two layers and prevents their separation. The calcified cartilage is less dense than the subchondral bone, so it acts as an intermediate layer, which softens the compression load on the cartilage and transfers it to the subchondral bone.
During the load, a complex distribution of three forces occurs-stretching, shear and compression. The articular matrix is deformed due to the expulsion of water (as well as metabolic products of cells) from the load zone, the concentration of ions in the interstitial fluid increases. The movement of water directly depends on the duration and strength of the applied load and is delayed by the negative charge of proteoglycans. During the deformation of the tissue, the proteoglycans are more closely pressed against each other, thereby effectively increasing the density of the negative charge, and the intermolecular repulsive negative charge of force in turn increases the resistance of the tissue of further deformation. Ultimately, the deformation reaches equilibrium, in which the external forces of the load are balanced by the internal forces of resistance-the swelling pressure (interaction of proteoglycans with ions) and mechanical stress (interaction of proteoglycans and collagens). When the load is eliminated, the cartilaginous tissue acquires its original shape by sucking water along with the nutrients. The initial (pre-load) tissue form is achieved when the swelling pressure of proteoglycans is balanced by the resistance of the collagen network to their spread.
Biomechanical properties of articular cartilage are based on the structural integrity of the tissue - the collagen-proteoglycan composition as a solid phase and water and dissolved in it ions as a liquid phase. Out of the load, the hydrostatic pressure of the articular cartilage is about 1-2 atm. This hydrostatic pressure can increase in vivo to 100-200 atm. In milliseconds during standing and up to 40-50 atm during walking. Studies in vitro have shown that the hydrostatic pressure of 50-150 atm (physiological) for a short period of time leads to a moderate growth of cartilage anabolism, for 2 hours - leads to loss of liquid cartilage, but not causing any other changes. The question remains how fast the chondrocytes react in vivo to this kind of load.
Induced reduction of hydration with subsequent increase in the concentration of proteoglycans leads to attraction of positively charged ions, such as H + and Na +. This leads to a change in the total ion composition and pH of the ECM, and chondrocytes. Prolonged loading induces a decrease in pH and a simultaneous decrease in the synthesis of proteoglycans by chondrocytes. Perhaps the influence of the extracellular ionic environment on synthetic processes is also partly related to its effect on the composition of the ECM. The newly synthesized molecules of aggrecan in a weakly acidic medium later than in normal conditions ripen into aggregated forms. It is likely that a decrease in pH around chondrocytes (for example, during a load) allows more newly synthesized aggrecane molecules to reach the interterritorial matrix.
When the load is eliminated, the water returns from the synovial cavity, carrying with it nutrients for the cells. In cartilage damaged by osteoarthritis, the concentration of proteoglycans is reduced, therefore, during the load, water moves not only vertically into the synovial cavity, but also in other directions, thereby reducing the supply of chondrocytes.
Immobilization or a small load leads to a marked decrease in synthetic processes in the cartilaginous tissue and the content of proteoglycans, while an increase in dynamic load leads to a moderate increase in the synthesis and content of proteoglycans. Tight training (20 km per day for 15 weeks) in dogs caused changes in the content of proteoglycans, in particular, a sharp decrease in their concentration in the surface zone. There has been some reversible softening of the cartilage and remodeling of the subchondral bone. A large static load, however, caused cartilage damage and subsequent degeneration. In addition, the loss of Aggrecan ECM initiates abnormal changes characteristic of osteoarthrosis. Loss of aggrecan leads to attraction of water and swelling of the remaining small amount of proteoglycans. This dissolution of aggrecan helps to reduce the density of local fixed charge and ultimately leads to a change in osmolarity.