Respiratory failure: causes and pathogenesis
Last reviewed: 23.04.2024
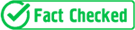
All iLive content is medically reviewed or fact checked to ensure as much factual accuracy as possible.
We have strict sourcing guidelines and only link to reputable media sites, academic research institutions and, whenever possible, medically peer reviewed studies. Note that the numbers in parentheses ([1], [2], etc.) are clickable links to these studies.
If you feel that any of our content is inaccurate, out-of-date, or otherwise questionable, please select it and press Ctrl + Enter.
Causes and mechanisms of ventilation and parenchymal respiratory failure
Respiratory failure occurs when any of the functional components of the respiratory system - lung parenchyma, thoracic wall, circulatory system in the small circle, the condition of the alveolar-capillary membrane, nervous and humoral regulation of respiration, are violated. Depending on the prevalence of these or other changes in the gas composition of the blood, two main forms of respiratory failure are distinguished: ventricular (hypercapnic) and parenchymal (hypoxemic), each of which can be acute or chronic.
Ventilating (hypercapnic) respiratory failure
Ventilation (hypercapnic) form of respiratory failure is characterized mainly by a total decrease in the volume of alveolar ventilation (alveolar hypoventilation) and minute volume of respiration (MOU), a decrease in CO2 excretion from the body and, correspondingly, development of hypercapnia (PaCO2> 50 mmHg), and then and hypoxemia.
The causes and mechanisms of the development of ventilation respiratory failure are closely related to the violation of the process of removing carbon dioxide from the body. As is known, the process of gas exchange in the lungs is determined by:
- level of alveolar ventilation;
- diffusion ability of the alveolar-capillary membrane in relation to O 2 and CO 2;
- the amount of perfusion;
- the ratio of ventilation and perfusion (ventilation-perfusion ratio).
From a functional point of view, all airway paths in the lungs are divided into conductive paths and a gas exchange (or diffusion) zone. In the field of the conducting pathways (in the trachea, bronchi, bronchioles and terminal bronchioles) during the inspiration, the air is progressively moved and mechanical mixing (convection) of fresh portions of atmospheric air with gas located in the physiological dead space before the next inspiration. Therefore this region received another name - convection zone. It is clear that the intensity of enrichment of the convection zone with oxygen and the reduction in the concentration of carbon dioxide is primarily determined by the intensity of pulmonary ventilation and the value of the minute volume of breathing (MOU).
Characteristically, as the approach to smaller generations of airway paths (from the 1 st to the 16 th generation) approaches, the translational motion of the air flow gradually slows down, and at the boundary of the convection zone completely ceases. This is due to a sharp increase in the total total cross-sectional area of each subsequent generation of bronchi and, respectively, with a significant increase in the overall resistance of small bronchi and bronchioles.
The subsequent generation of airways (from 17th to 23rd), including respiratory bronchioles, alveolar courses, alveolar sacs and alveoli, belong to the gas exchange (diffusion) zone, in which diffusion of gases through the alveolar-capillary membrane is carried out. In the diffusion zone "macroscopic" days | blue gas, both during respiratory movements, and during coughing is completely absent (V. Yu. Shanin). Gas exchange is carried out here only due to the molecular process of diffusion of oxygen and carbon dioxide. At the same time, the rate of molecular movement of CO2 - from the convection zone, through the entire diffusion zone to the alveoli and capillaries, and also from the alveoli to the convection zone - is determined by three main factors:
- gradient of the partial pressure of gases at the boundary of the convection and diffusion zones;
- ambient temperature;
- diffusion coefficient for a given gas.
It is important to note that the level of pulmonary ventilation and MOD almost does not affect the process of moving molecules of CO2 and O2 directly in the diffusion zone.
It is known that the diffusion coefficient of carbon dioxide is approximately 20 times higher than that of oxygen. This means that the diffusion zone does not create a big obstacle to carbon dioxide, and its exchange is almost completely determined by the state of the convection zone, i.e. Intensity of respiratory movements and the magnitude of MOD. With a total reduction in ventilation and a minute volume of breathing, the "washout" of carbon dioxide from the convection zone ceases, and its partial pressure increases. As a result, the pressure gradient of CO 2 at the boundary of the convection and diffusion zones decreases, the intensity of its diffusion from the capillary bed into the alveoli drops sharply, and hypercapnia develops.
In other clinical situations (for example, with parenchymal respiratory failure), when at a certain stage of the development of the disease there is a pronounced compensatory unit for the hyperventilation of undamaged alveoli, the rate of "washing away" of carbon dioxide from the convection zone increases significantly, which leads to an increase in the pressure gradient of CO 2 at the convection and diffusion zones and enhanced removal of carbon dioxide from the body. As a result, hypocapnia develops.
In contrast to carbon dioxide, the exchange of oxygen in the lungs and the partial pressure of carbon dioxide in the arterial blood (PaO 2 ) depend primarily on the functioning of the diffusion zone, in particular on the diffusion coefficient of O 2 and the state of capillary blood flow (perfusion), and the level ventilation and the state of the convection zone affect these indicators only to a small extent. Therefore, with the development of ventilation respiratory failure against the background of a total decrease in the minute volume of respiration, hypercapnia first arises and only then (usually at later stages of development of respiratory failure) is hypoxemia.
Thus, the ventilation (hypercapnic) form of respiratory failure indicates the incompetence of the "breathing pump". It can be caused by the following reasons:
- Disorders of central regulation of respiration:
- edema of the brain, exciting its stem divisions and the area of the respiratory center;
- stroke;
- craniocerebral trauma;
- neuroinfection;
- toxic effects on the respiratory center;
- hypoxia of the brain, for example, in severe heart failure;
- overdose of drugs that depress the respiratory center (narcotic analgesics, sedatives, barbiturates, etc.).
- Damage to the device that provides respiratory movements of the chest, i.e. Violations of the functioning of the so-called "pectoral furs" (peripheral nervous system, respiratory muscles, thorax):
- deformations of the chest (kyphosis, scoliosis, kyphoscoliosis, etc.);
- fractures of the ribs and spine;
- thoracotomy;
- a violation of the function of peripheral nerves (mainly diaphragmatic - Guillain-Barre syndrome, poliomyelitis, etc.);
- disorders of neuromuscular transmission (myasthenia gravis);
- fatigue or atrophy of the respiratory muscles against the background of a prolonged intensive cough, airway obstruction, restrictive breathing disorders, prolonged ventilation, etc.);
- a decrease in the efficiency of the diaphragm (for example, when it is flattened).
- Restrictive respiratory disorders, accompanied by a decrease in MOD:
- pronounced pneumothorax;
- massive pleural effusion;
- interstitial diseases of the lungs;
- total and subtotal pneumonia, etc.
Thus, most of the causes of ventilation respiratory failure are associated with violations of extrapulmonary respiration and its regulation (CNS, thorax, respiratory muscles). Among the "pulmonary" mechanisms of ventilation respiratory failure, restrictive breathing disorders, caused by a decrease in the ability of the lungs, chest or pleura to spread during inspiration, are of primary importance. Restrictive disorders develop in many acute and chronic diseases of the respiratory system. In this connection, within the framework of ventilation respiratory failure, a special restrictive type of respiratory failure is distinguished, most often due to the following reasons:
- diseases of the pleura that limit the excursion of the lung (exudative pleurisy, hydrothorax, pneumothorax, fibrotorax, etc.);
- a decrease in the volume of the functioning parenchyma of the lung (atelectasis, pneumonia, resection of the lung, etc.);
- inflammatory or hemodynamically caused by infiltration of pulmonary tissue, leading to an increase in the "stiffness" of the pulmonary parenchyma (pneumonia, interstitial or alveolar pulmonary edema in left ventricular heart failure, etc.);
- pneumosclerosis of various etiologies, etc.
It should also be taken into account that the cause of hypercapnia and ventilation respiratory failure can be any pathological processes, accompanied by a total decrease in alveolar ventilation and a minute volume of respiration. Such a situation can arise, for example, with severe airway obstruction (bronchial asthma, chronic obstructive bronchitis, emphysema, dyskinesia of the membrane part of the trachea, etc.), with a significant decrease in the volume of functioning alveoli (atelectasis, interstitial lung diseases, etc.) or with considerable fatigue and atrophy of the respiratory muscles. Although in all these cases other pathophysiological mechanisms (violations of gas diffusion, ventilation-perfusion ratios, capillary blood flow of the lungs, etc.) also participate in the onset of respiratory failure. In these cases, it is usually about the formation of mixed ventilation and parenchymal) respiratory failure.
It should also be added that in case of acute ventilation respiratory failure RaCO2 increase is usually accompanied by a decrease in blood pH and development of respiratory acidosis due to a decrease in the ratio of HCO3 / H2CO3, which, as is known, determines the pH value. With chronic respiratory failure of ventilation type, such a pronounced decrease in pH due to compensatory increase in the concentration and carbonates in serum does not occur.
1. Ventilation (hypercapnic) respiratory failure is characterized by:
- total alveolar hypoventilation and a decrease in the minute volume of respiration,
- hypercapnia,
- hypoxemia (at later stages of formation of respiratory failure),
- signs of compensated or decompensated respiratory acidosis.
2. The main mechanisms for the development of ventilation (hypercapnic) forms of respiratory failure:
- disturbed central regulation of breathing;
- damage to the device providing respiratory motion of the chest (peripheral nerves, respiratory muscles, chest wall);
- marked restrictive disorders, accompanied by a decrease in MOU.
Parenchymal respiratory failure
The parenchymal (hypoxemic) form of respiratory failure is characterized by a significant disruption of the process of oxygenation of blood in the lungs, which leads to the predominant pinging of PaO2 in the arterial blood - hypoxemia.
The main mechanisms of the development of hypoxemia in the parenchymal form of respiratory failure:
- violation of ventilation-perfusion relations (\ / 0) with the formation of right-heart "shunting" of the blood (alveolar shunt) or an increase in the alveolar dead space;
- a decrease in the total functioning surface of the alveolar-capillary membranes;
- diffusion of gases.
Violation of ventilation-perfusion relations
The emergence of hypoxemic respiratory failure in many diseases of the respiratory system is most often caused by a violation of ventilation-perfusion relations. Normally, the ventilation-perfusion ratio is 0.8 1.0. There are two possible violations of these relationships, each of which can lead to the development of respiratory failure.
Local hypoventilation of alveoli. In this variant of parenchymal respiratory failure, hypoxemia occurs if a rather intensive blood flow continues through poorly ventilated or unventilated alveoli. The ratio of ventilation and perfusion values here is reduced by V / Q <0.8), which results in the discharge of venous blood not sufficiently oxygenated in these sections of the lung to the left heart and large circulatory (venous bypass). This causes a decrease in the partial pressure of O 2 in the arterial blood - hypoxemia.
If there is no ventilation in such a section with a preserved blood flow, the V / Q ratio approaches zero. It is in these cases that a right-left-handed alveolar shunt is formed, through which the non-oxygenated venous blood is "transferred" to the left heart and aorta, reducing PAO 2 in the arterial blood. This mechanism develops hypoxemia in obstructive pulmonary diseases, pneumonia, pulmonary edema and other diseases, accompanied by uneven (local) reduction of alveolar ventilation and the formation of venous bypass grafting. In this case, unlike ventilating respiratory failure, the total minute ventilation volume does not decrease for a long time, and even a tendency to hyperveptic lungs is observed.
It should be emphasized that in the early stages of development of parenchymal respiratory failure, hypercapnia does not develop, since pronounced hyperventilation of intact alveoli accompanied by intensive removal of CO 2 from the body completely compensates local disturbances of CO 2 metabolism . Moreover, with pronounced hyperventilation of undamaged alveoli, hypocapnia appears, which in itself exacerbates respiratory distress.
This is primarily due to the fact that hypocapnia reduces the body's adaptation to hypoxia. As is known, a decrease in PaCO2 in the blood shifts the hemoglobin dissociation curve to the left, which increases the affinity of hemoglobin for oxygen and reduces the release of O 2 in peripheral tissues. Thus, hypocapnia, arising at the initial stages of parenchymal respiratory failure, additionally increases the oxygen starvation of peripheral organs and tissues.
In addition, a decrease in PACO 2 reduces the afferent impulses of the receptors of the carotid sinus and the medulla oblongata and reduces the activity of the respiratory center.
Finally, hypocapnia changes the ratio of bicarbonate and carbon dioxide in the blood, which leads to an increase in HCO3 / H2SO3 and pH and the development of respiratory alkalosis (in which the vessels spasmodic and the blood supply of vital organs worsens).
It should be added that in the late stages of development of parenchymal respiratory failure, not only oxygenation of the blood, but also ventilation of the lungs (for example, due to fatigue of the respiratory musculature or increase in lung stiffness due to inflammatory edema) is violated, and hypercapnia appears reflecting the formation of a mixed form of respiratory failure combining in itself signs of parenchymal and ventilation respiratory failure.
The most frequent parenchymal respiratory failure and a critical reduction in the ventilation-perfusion ratio develop in lung diseases accompanied by local (uneven) hypoventilation of the alveoli. There are a lot of such diseases:
- chronic obstructive pulmonary diseases (chronic obstructive bronchitis, bronchiolitis, bronchial asthma, cystic fibrosis, etc.);
- central lung cancer;
- pneumonia;
- pulmonary tuberculosis, etc.
With all these diseases, obstruction of the airways due to uneven inflammatory infiltration and pronounced edema of bronchial mucosa (bronchitis, bronchiolitis), to an increase in the amount of viscous secretion (sputum) in the bronchial tubes (bronchitis, bronchiolitis, bronchiectasis, pneumonia, etc.) is to some extent or other. , spasm of smooth muscles of small bronchi (bronchial asthma), early expiratory closure (collapse) of small bronchi (the most pronounced in patients with emphysema of the lungs), deformation and compression of the bronchial tubes olyu, foreign body, etc. Therefore, it is advisable to allocate a special obstructive type of respiratory failure due to air flow violation along large and / or small airways, which in most cases is considered in the framework of parenchymal respiratory failure. At the same time, with severe airway obstruction, in some cases, pulmonary ventilation and MOD are significantly reduced, and ventilation (more precisely - mixed) respiratory failure develops.
Increased alveolar dead space. Another option for changing ventilation-perfusion ratios is associated with local impairment of pulmonary blood flow, for example, in thrombosis or embolism of the pulmonary artery branches. In this case, despite the maintenance of normal ventilation of the alveoli, the perfusion of the limited area of the lung tissue is sharply reduced (V / Q> 1.0) or completely absent. There is the effect of a sudden increase in functional dead space, and if its volume is sufficiently large, hypoxemia develops. In this case, a compensatory increase in the concentration of CO2 in the air exhaled from the normally perfused alveoli occurs, which usually completely neutralizes the violation of the exchange of carbon dioxide in the non - perfused alveoli. In other words, this variant of parenchymal respiratory failure is also not accompanied by an increase in the partial pressure of CO 2 in the arterial blood.
Parenchymal respiratory failure by the mechanism of increasing the alveolar dead space and V / Q values. Most often develops with the following diseases:
- Thromboembolism of the branches of the pulmonary artery.
- Respiratory distress syndrome of adults.
Reduction of the functioning surface of the alveolar-capillary membrane
With pulmonary emphysema, interstitial lung fibrosis, compression atelectasis and other diseases, oxygenation of the blood may decrease as a result of a decrease in the total functioning surface of the alveolar-capillary membrane. In these cases, as with other variants of parenchymal respiratory failure, the change in the gas composition of the blood is primarily manifested by arterial hypoxemia. In later stages of the disease, for example, with fatigue and atrophy of the respiratory muscles, hypercapnia may develop.
Diffusion of gases
The diffusion coefficient of oxygen is relatively low, its diffusion is disturbed in many lung diseases accompanied by inflammatory or hemodynamic edema of the interstitial tissue and an increase in the distance between the inner surface of the alveolus and the capillary (pneumonia, interstitial lung diseases, pneumosclerosis, hemodynamic pulmonary edema in left ventricular heart failure, etc.). . In most cases, the disturbance of blood oxygenation in the lungs is due to other pathophysiological mechanisms of development of respiratory failure (for example, a decrease in ventilation-perfusion ratios), and a decrease in the diffusion rate of O 2 only aggravates it.
Since the diffusion rate of CO 2 is 20 times higher than that of O 2, the transfer of carbon dioxide through the alveolar-capillary membrane can be disturbed only if it is significantly thickened or with a widespread lesion of the lung tissue. Therefore, in most cases, a violation of the diffusive capacity of the lungs increases only hypoxemia.
- Parenchymal (hypoxemic) respiratory failure in most cases is characterized by:
- uneven local alveolar hypoventilation without a reduction in the overall index of MOD,
- pronounced hypoxemia,
- at the initial stage of the formation of respiratory failure - hyperventilation of intact alveoli, accompanied by hypocapnia and respiratory alkalosis,
- at the later stages of the formation of respiratory failure - the addition of ventilation disorders, accompanied by hypercapnia and respiratory or metabolic acidosis (stage of mixed respiratory failure).
- The main mechanisms of development of the parenchymal (hypoxemic) form of respiratory failure:
- violation of ventilation-perfusion relations in obstructive type of respiratory failure or lesion of the capillary bed of the lungs,
- a decrease in the total functioning surface of the alveolar-capillary membrane,
- diffusion of gases.
The distinction between the two forms of respiratory failure (ventilation and parenchymal) is of great practical importance. When treating the ventilation form of respiratory failure, respiratory support is most effective, allowing to restore the reduced minute volume of breathing. On the contrary, in the parenchymal form of respiratory failure, hypoxemia is caused by a violation of ventilation-perfusion ratios (for example, by the formation of venous "shunting" of the blood), so oxygen inhalation therapy, even in high concept (high FiO2), is ineffective. Poorly helps with this and the artificial increase in MOU (for example, with the help of ventilation). Stable improvement in parenchymal respiratory failure can be achieved only by an adequate correction of ventricio-perfusion relations and elimination of some other mechanisms of development of this form of respiratory failure.
Almost clinically-instrumental verification of obstructive and restrictive types of respiratory failure is also important, since it allows choosing the optimal tactics for managing patients with respiratory failure.
In clinical practice, there is often a mixed version of respiratory failure, accompanied by both a violation of oxygenation of the blood (hypoxemia), and total alveolar hypoventilation (hypercapnia and hypoxemia). For example, in severe pneumonia, ventilation-perfusion relationships are violated and the alveolar shunt is formed, therefore, PaO2 decreases, and hypoxemia develops. Massive inflammatory infiltration of pulmonary tissue is often accompanied by a significant increase in lung rigidity, resulting in alveolar ventilation, the rate of "washing away" of carbon dioxide is reduced, and hypercapnia develops.
Progressive ventilation disorders and the development of hypercapnia are also facilitated by the expressed fatigue of the respiratory muscles and the restriction of the volume of respiratory movements upon the appearance of pleural pain.
On the other hand, with some restrictive diseases accompanied by ventilation respiratory insufficiency and hypercapnia, sooner or later violations of bronchial patency develop, ventilation-perfusion relations decrease, and a parenchymal component of respiratory failure accompanied by hypoxemia joins. Nevertheless, in any case it is important to assess the prevailing mechanisms of respiratory failure.
Violations of the acid-base state
Different forms of respiratory failure may be accompanied by a violation of the acid-base state, which is more typical for patients with acute respiratory failure, including those that developed against a background of long-standing chronic respiratory failure. It is in these cases that decompensated respiratory or metabolic acidosis or respiratory alkalosis develops, which significantly aggravates respiratory failure and contributes to the development of severe complications.
Mechanisms for maintaining the acid-base state
The acid-base state is the ratio of the concentrations of hydrogen (H + ) and hydroxyl (OH - ) ions in the internal environment of the organism. Acidic or alkaline reaction of the solution depends on the content of hydrogen ions in it, the indicator of this content is the pH value, which is the negative decimal logarithm of the molar concentration of H + ions :
PH = - [H + ].
This means, for example, that at pH = 7.4 (neutral reaction of the medium), the concentration of H + ions , ie [H + ], is 10 -7.4 mmol / l. When the acidity of the biological medium increases, its pH decreases, and when the acidity decreases, it increases.
The pH value is one of the most "hard" parameters of the blood. His fluctuations in the norm are extremely insignificant: from 7.35 to 7.45. Even small deviations of pH from the normal level towards decreasing (acidosis) or increasing (alkalosis) lead to a significant change in the redox processes, the activity of the riments, the permeability of the cell membranes, and to other disorders that are fraught with dangerous consequences for the vital activity of the organism.
The concentration of hydrogen ions is almost completely determined by the ratio of bicarbonate and carbon dioxide:
HCO3 - / H 2 CO 3
The content of these substances in the blood is closely related to the process of blood transfer of carbon dioxide (CO 2 ) from tissues to the lungs. Physically dissolved CO 2 diffuses from the tissues into the erythrocyte, where the hydration of the molecule (CO 2 ) takes place under the action of the enzyme carbonic anhydrase to form carbonic acid H 2 CO 3, immediately dissociating to form hydrogen bicarbonate (HCO 3- ) ions (H + ):
CO 2 + H 2 O ↔ H 2 CO 3 ↔ HCO 3- + H +
Part accumulated in erythrocytes ion HCO 3, according to the concentration gradient out to the plasma. In the ion exchange HCO 3- to erythrocytes we arrive chlorine (C1 - ), whereby the equilibrium distribution of electric charges breaks.
H + ions , formed during the dissociation of carbon dioxide, are attached to the molecule of myoglobin. Finally, some of the CO 2 can be bound by directly attaching the hemoglobin protein component to the amino groups to form a carbamic acid residue (NNCOOH). Thus, in the blood flowing from the tissue of 27% CO2 is transferred in the form of bicarbonate (HCO 3 ) in erythrocytes, 11% CO 2 forms a carbamic compound to hemoglobin (karbogemoglobin), about 12% CO 2 remains in dissolved form or in form of nondissociated carbonic acid (H2CO3), and the remaining amount of CO 2 (about 50%) is dissolved as HCO 3- in the plasma.
Normally, the concentration of bicarbonate (HCO 3- ) in the blood plasma is 20 times higher than that of carbon dioxide (H2CO3). It is at this ratio of HCO 3 and H2CO3 that the normal pH is maintained at 7.4. If the concentration of bicarbonate or carbon dioxide varies, their ratio changes, and the pH shifts to acid (acidosis) or alkaline (alkalosis) side. In these conditions, the normalization of pH requires the connection of a number of compensatory regulatory mechanisms restoring the previous ratio of acids and bases in the blood plasma, as well as in various organs and tissues. The most important of these regulatory mechanisms are:
- Buffer systems of blood and tissues.
- Change in ventilation.
- Mechanisms of renal regulation of the acid-base state.
Buffer systems of blood and tissues consist of acid and a conjugated base.
When interacting with acids, the latter are neutralized by the alkaline component of the buffer, upon contact with bases their excess is bound to the acid component.
The bicarbonate buffer has an alkaline reaction and consists of a weak carbonic acid (H2CO3) and its sodium salt - sodium bicarbonate (NaHCO3) as the conjugate base. When interacting with an acid, the alkaline component of bicarbonate buffer (TaHCO3) neutralizes it with the formation of H2CO3, which dissociates into CO 2 and H 2 O. Excess is removed with exhaled air. When interacting with bases, the acid component of the buffer (H2CO3) is bound by an excess of bases to form bicarbonate (HCO 3- ), which is then released by the kidneys.
Phosphate buffer consists of monobasic sodium phosphate (NaH2PO4), which plays the role of acid, and dibasic sodium phosphite (NaH2PO4), acting as a conjugate base. The principle of this buffer is the same as that of bicarbonate, but its buffer capacity is low, since the phosphate content in the blood is low.
Protein buffer. The buffer properties of plasma proteins (albumin, etc.) and hemoglobin of erythrocytes are due to the fact that the amino acids in their composition contain both acid (COOH) and basic (NH 2 ) groups, and can dissociate with the formation of both hydrogen and hydroxyl ions depending on the reaction of the medium. Most of the buffer capacity of the protein system accounts for the proportion of hemoglobin. In the physiological pH range, oxyhemoglobin is a stronger acid than deoxyhemoglobin (reduced hemoglobin). Therefore, releasing oxygen in the tissues, the reduced hemoglobin acquires a higher ability to bind H + priests . When oxygen is absorbed in the lungs, hemoglobin acquires the properties of acid.
Buffer properties of blood are due, in fact, the total effect of all anionic groups of weak acids, the most important of which are bicarbonates and anionic groups of proteins ("proteinates"). These anions, which have buffer effects, are called buffer bases (BB).
The total concentration of the buffer bases of the blood is about <18 mmol / L and does not depend on the shifts in the blood pressure of CO 2. Indeed, by increasing the pressure S0O 2 blood formed equal amounts of H + and HCO 3. Proteins bind H + ions, which leads to a decrease in the concentration of "free" proteins, which have buffer properties. At the same time, the bicarbonate content increases by the same amount, and the total concentration of buffer bases remains the same. Conversely, as the pressure of CO2 in the blood decreases, the content of proteinates increases and the concentration of bicarbonate decreases.
If in the blood the content of nonvolatile acids changes (lactic acid in hypoxia, acetoacetic and beta-oxymosphate in diabetes mellitus, etc.). The total concentration of buffer bases will be different from normal.
The deviation of buffer bases from the normal level (48 mmol / l) is called base excess (BE); in the norm it is zero. With a pathological increase in the number of buffer bases, BE becomes positive, and with a decrease in negative. In the latter case, it is more correct to use the term "deficiency of bases".
The BE index allows one to judge, therefore, about the shifts in the "reserves" of buffer bases when the content of nonvolatile acids in the blood changes, and even the latent (compensated) shifts in the acid-base state can be diagnosed.
The change in pulmonary ventilation is the second regulatory mechanism ensuring a constant pH of the blood plasma. When blood passes through the lungs in erythrocytes and blood plasma, there are reactions, reverse to those described above:
H + + HCO 3 -H2CO3 ↔ CO2 + H2O.
This means that when the CO 2 is removed from the blood, the equivalent number of H + ions disappears in it . Consequently, breathing plays a very important role in maintaining the acid-base state. So, if as a result of metabolic disorders in tissues the acidity of the blood increases and the state of moderate metabolic (non-respiratory) acidosis develops, the intensity of pulmonary ventilation (hyperventilation) increases in the reflex (respiratory center). The result »» removes a large amount of CO2 and, accordingly, hydrogen ions (H + ), due to which the pH returns to the initial level. Conversely, an increase in the content of bases (metabolic non-respiratory alkalosis) is accompanied by a decrease in the intensity of ventilation (hypoventilation), the pressure of CO 2 and the concentration of H + ions increase, and the pH shift to the alkaline side is compensated.
The role of the nights. The third regulator of the acid-base state is the kidneys, which remove the H + ions from the body and reabsorb sodium bicarbonate (NaHCO3). These important processes are carried out mainly in the renal tubules. Three main mechanisms are used:
Exchange of hydrogen ions on sodium ions. This process is based on the reaction activated by carbonic anhydrase: CO 2 + H 2 O = H 2 CO 3; The resulting carbon dioxide (H2CO3) dissociates into H + and HCO 3 ions . Ions are released into the lumen of the tubules, and an equivalent amount of sodium ions (Na + ) is supplied from the tubular fluid . As a result, the body is freed from hydrogen ions and at the same time replenishes the reserves of sodium bicarbonate (NaHCO3), which is reabsorbed into the interstitial tissue of the kidney and enters the bloodstream.
Acidogenesis. Similarly, the ion exchange of H + with Na + ions occurs with the participation of dibasic phosphate. The hydrogen ions released into the lumen of the tubule are bound by the anion HOP4 2- with formation of monobasic sodium phosphate (NaH2PO4). Simultaneously, an equivalent amount of Na + ions enters the epithelial cell of the tubule and binds to the HCO 3- ion to form Na + (NaHCO3) bicarbonate . The latter is reabsorbed and enters the bloodstream.
Ammoniogenesis occurs in the distal renal tubules, where ammonia is formed from glutamine and other amino acids. Last neutralizes HCl urine and binds hydrogen ions to form Na + and C1 -. Reabsorbing sodium in conjunction with the ion HCO 3- also forms sodium bicarbonate (NaHCO3).
Thus, in the tubular fluid, most of the H + ions coming from the tubular epithelium bind to HCO 3-, HPO4 2- ions and are excreted in the urine. At the same time, an equivalent amount of sodium ions enters the tubule cells to form sodium bicarbonate (NaHCO3), which is reabsorbed in the tubules and replenishes the alkaline component of the bicarbonate buffer.
Main indicators of acid-base state
In clinical practice, the following indices of arterial blood are used to evaluate the acid-base state:
- The pH of the blood is the value of the negative decimal logarithm of the molar concentration of H + ions . The pH of the arterial blood (plasma) at 37 ° C varies within narrow limits (7.35-7.45). Normal pH does not mean that there is no disturbance of the acid-base state and can occur in so-called compensated variants of acidosis and alkalosis.
- PaCO 2 is the partial pressure of CO 2 in the arterial blood. The normal values of Raco 2 are 35-45 mm, Hg. Art. In men and 32-43 mm Hg. Art. Among women.
- Buffer bases (BB) - the sum of all blood anions that have buffer properties (mainly bicarbonates and protein ions). The normal value of explosive is an average of 48.6 mol / l (from 43.7 to 53.5 mmol / l).
- Standard bicarbonate (SВ) - the content of the bicarbonate ion in the plasma. Normal values for men - 22,5-26,9 mmol / l, for women - 21,8-26,2 mmol / l. This indicator does not reflect the buffer effect of proteins.
- Excess bases (BE) - the difference between the actual value of buffer base content and their normal value (the normal value is from-2.5 to 2.5 mmol / l). In capillary blood, the values of this indicator are from -2.7 to +2.5 in men and from -3.4 to +1.4 in women.
In clinical practice, usually used 3 indicators of the acid-base state: pH, PaCO 2 and BE.
Changes in the acid-base state in respiratory failure
In many pathological conditions, including with respiratory insufficiency, so many acids or bases can accumulate in the blood that the above-described regulatory mechanisms (blood buffer systems, respiratory and excretory systems) can no longer maintain the pH at a constant level, and acidosis develops or alkalosis.
- Acidosis is a violation of the acid-base state, in which an absolute or relative excess of acids appears in the blood and the concentration of hydrogen ions increases (pH <7.35).
- Alkalosis is characterized by an absolute or relative increase in the number of bases and a decrease in the concentration of hydrogen ions (pH> 7.45).
According to the mechanisms of occurrence, there are 4 types of violations of the acid-base state, each of which can be compensated and decompensated:
- respiratory acidosis;
- respiratory alkalosis;
- non-respiratory (metabolic) acidosis;
- non-respiratory (metabolic) alkalosis.
Aspirate acidosis
Respiratory acidosis develops with severe total violations of pulmonary ventilation (alveolar hypoventilation). These changes in the acid-base state are based on an increase in the partial pressure of CO 2 in the arterial blood of PaCO 2 ).
With compensated respiratory acidosis, the pH of the blood does not change due to the action of the compensatory mechanisms described above. The most important of them are 6carbonate and protein (hemoglobin) buffer, as well as a renal mechanism for the release of H + ions and sodium bicarbonate retention (NaHCO3).
In the case of hypercapnia (ventilation) respiratory failure, the mechanism of strengthening pulmonary ventilation (hyperventilation) and removing H + and CO2 ions with respiratory acidosis is of no practical importance, since in such patients there is by definition primary pulmonary hypoventilation due to severe pulmonary or extrapulmonary pathology. It is accompanied by a significant increase in the partial pressure of CO2 in the blood - hypercapia. In connection with the effective action of buffer systems and, especially, as a result of the incorporation of the renal compensatory mechanism of sodium bicarbonate retention, the content of standard bicarbonate (SB) and excess bases (BE) is increased in patients.
Thus, compensated respiratory acidosis is characterized by:
- Normal blood pH values.
- Increasing the partial pressure of C0 2 in the blood (RaS0 2 ).
- Increase in standard bicarbonate (SB).
- Increase in excess bases (BE).
The depletion and inadequacy of the mechanisms of compensation leads to the development of decompensated respiratory acidosis, in which the pH of the plasma decreases below 7.35. In some cases, the levels of standard bicarbonate (SB) and excess bases (BE) also decrease to normal values, indicating depletion of base stocks.
Respiratory alkalosis
It was shown above that parenchymal respiratory failure in some cases is accompanied by hypocapnia due to the pronounced compensatory hyperventilation of undamaged alveoli. In these cases, respiratory alkalosis develops as a result of increased elimination of carbon dioxide in the event of disturbance of the external respiration of a hyperventilation type. As a result, the ratio of HCO3 - / H2CO3 increases and, accordingly, the pH of the blood increases.
Compensation for respiratory alkalosis is possible only against the background of chronic respiratory failure. Its main mechanism is a decrease in the secretion of hydrogen ions and inhibition of the reabsorption of bicarbonate in the renal tubules. This leads to a compensatory decrease in the standard bicarbonate (SB) and to the deficiency of bases (negative BE).
Thus, compensated respiratory alkalosis is characterized by:
- Normal blood pH value.
- Significant decrease in pCO2 in the blood.
- Compensatory reduction of standard bicarbonate (SB).
- Compensatory deficiency of bases (negative value of BE).
When respiratory alkalosis is decompensated, the blood pH increases, and previously lowered SB and BE values can reach normal values.
Nonspiratory (metabolic) acidosis
Nonspiratory (metabolic) acidosis is the most severe form of acid-base disorder, which can develop in patients with very severe respiratory failure, pronounced hypoxemia of blood and hypoxia of organs and tissues. The mechanism of development of non-respiratory (metabolic) acidosis in this case is associated with the accumulation in the blood of so-called nonvolatile acids (lactic acid, beta-oksymaslyana, acetoacetic, etc.). Recall that in addition to severe respiratory failure, the causes of non-respiratory (metabolic) acidosis can be:
- Expressed disorders of tissue metabolism in decompensated diabetes mellitus, prolonged starvation, thyrotoxicosis, fever, hypoxia, organon on the background of severe heart failure and so-called.
- Kidney disease, accompanied by a primary lesion of the renal tubules, leading to a violation of the excretion of hydrogen ions and the reabsorption of sodium bicarbonate (renal tubular acidosis, renal failure, etc.)
- Loss of the body of a large number of bases in the form of bicarbonates with digestive juices (diarrhea, vomiting, stenosis of the pylorus, surgical interventions). Acceptance of certain drugs (ammonium chloride, calcium chloride, salicylates, inhibitors of carbonic anhydrase, etc.).
With compensated non-respiratory (metabolic) acidosis, the bicarbonate blood buffer is included in the compensation process, which binds the acids accumulating in the body. A decrease in sodium bicarbonate results in a relative increase in the concentration of carbonic acid (H2CO3), which dissociates into H2O and CO2. H + ions bind to proteins, mainly hemoglobin, in connection with which from the erythrocytes, in exchange for the hydrogen cations entering there, Na +, Ca2 + and K + leave .
Thus, compensated metabolic acidosis is characterized by:
- Normal blood pH.
- Reduction of standard bicarbonates (BW).
- Deficiency of buffer bases (negative value of BE).
The depletion and insufficiency of the compensatory mechanisms described lead to the development of decompensated non-respiratory (metabolic) acidosis, in which the pH of the blood drops to less than 7.35.
Non-respiratory (metabolic) alkalosis
Nonspiratory (metabolic) alkalosis with respiratory failure is not typical.
Other complications of respiratory failure
Changes in the gas composition of the blood, acid-base state, as well as violations of pulmonary hemodynamics in severe cases of respiratory failure lead to severe complications about the side of other organs and systems, including the brain, heart, kidneys, gastrointestinal tract, vascular system, etc. .
For acute respiratory failure, relatively quickly developing severe systemic complications are more common, mainly due to the pronounced hypoxia of the tissues, leading to disturbances in the metabolic processes that occur in them and the functions they perform. The occurrence of multi-organ failure in the context of acute respiratory failure significantly increases the risk of an unfavorable outcome of the disease. Below is a rather incomplete list of systemic complications of respiratory failure:
- Cardiac and vascular complications:
- myocardial ischemia;
- arrhythmia of the heart;
- decrease in stroke volume and cardiac output;
- arterial hypotension;
- thrombosis of deep veins;
- PE.
- Neuromuscular complications:
- stupor, sopor, coma;
- psychosis;
- delirium;
- polyneuropathy of the critical condition;
- contractures;
- muscle weakness.
- Infectious complications:
- sepsis;
- abscess;
- nosocomial pneumonia;
- pressure sores;
- other infections.
- Gastrointestinal complications:
- acute stomach ulcer;
- gastrointestinal bleeding;
- liver damage;
- malnutrition;
- complications of enteral and parenteral nutrition;
- acuminate cholecystitis.
- Kidney complications:
- acute renal insufficiency;
- electrolyte disturbances, etc.
It should also be taken into account the possibility of developing complications associated with the presence of a tracheal intubation tube in the lumen of the trachea, as well as with the ventilation.
In chronic respiratory failure, the severity of systemic complications is significantly less than in acute respiratory failure, and the formation of 1) pulmonary arterial hypertension and 2) chronic pulmonary heart is the foreground.
Pulmonary arterial hypertension in patients with chronic respiratory failure is formed under the influence of several pathogenetic mechanisms, the main one of which is chronic alveolar hypoxia, leading to the occurrence of hypoxic pulmonary vasoconstriction. This mechanism is known as the Euler-Lilestride reflex. As a result of this reflex, the local pulmonary blood flow adapts to the level of pulmonary ventilation intensity, so the ventilation-perfusion relations are not violated or become less pronounced. However, if alveolar hypoventilation is expressed to a large extent and extends to large areas of lung tissue, a generalized increase in the tone of pulmonary arterioles develops, leading to an increase in total pulmonary vascular resistance and the development of pulmonary arterial hypertension.
The formation of hypoxic pulmonary vasoconstriction is also facilitated by hypercapnia, bronchial obstruction and endothelial dysfunction. Anatomical changes in the pulmonary vascular bed play an important role in the onset of pulmonary arterial hypertension: compression and desolation of arterioles and capillaries due to gradually progressing pulmonary fibrosis and pulmonary emphysema, thickening of the vascular wall behind the account of hypertrophy of muscular cells of a medicine, development in conditions of a chronic disturbance of a blood flow and above hydrochloric mikrotrombozov platelet aggregation, recurrent thromboembolism small branches of the pulmonary artery, and others.
Chronic pulmonary heart develops in all cases of long-term lung diseases, chronic respiratory failure, progressive pulmonary arterial hypertension. But modern concepts, the long process of formation of the chronic pulmonary heart involves the appearance of a number of structural and functional changes in the right heart, the most significant of which are myocardial hypertrophy of the right ventricle and atrium, widening of their cavities, cardiofibrosis, diastolic and systolic dysfunction of the right ventricle, insufficiency of the tricuspid valve, increased CVP, stagnant phenomena in the venous channel of the great circle of blood circulation. These changes are caused by the formation of pulmonary pulmonary hypertension in chronic respiratory failure, persistent or transient increase in postnagruzka on the right ventricle, an increase in intramyocardial pressure, as well as activation of tissue neurohormonal systems, cytokine release, and development of endothelial dysfunction.
Depending on the absence or presence of signs of right ventricular heart failure, a compensated and decompensated chronic pulmonary heart is isolated.
For acute respiratory failure, the most common occurrence of systemic complications (cardiac, vascular, renal, neurologic, gastrointestinal, etc.), which significantly increases the risk of an unfavorable outcome of the disease. For chronic respiratory failure, the gradual development of pulmonary hypertension and chronic pulmonary heart is more characteristic.