Neural stem cells
Last reviewed: 23.04.2024
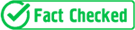
All iLive content is medically reviewed or fact checked to ensure as much factual accuracy as possible.
We have strict sourcing guidelines and only link to reputable media sites, academic research institutions and, whenever possible, medically peer reviewed studies. Note that the numbers in parentheses ([1], [2], etc.) are clickable links to these studies.
If you feel that any of our content is inaccurate, out-of-date, or otherwise questionable, please select it and press Ctrl + Enter.
Experimental evidence of the possibility of regeneration of CNS cells was obtained much earlier than the discovery of embryonic stem cells in studies showing the presence in the neocortex, hippocampus and olfactory bulbs of the brain of adult rat cells that capture 3H-thymidine, that is, capable of protein synthesis and fission. Back in the 60s of the last century it was assumed that these cells are the precursors of neurons and take a direct part in the learning and memory processes. A little later, synapses were detected on de novo neurons, and the first works on the use of embryonic stem cells for the induction of neuronogenesis in vitro appeared. At the end of the 20th century, experiments with directed differentiation of ESC into neural progenitor cells, dopaminergic and serotonergic neurons led to a revision of the classical ideas about the ability of mammalian nerve cells to regenerate. The results of numerous studies have convincingly proved both the reality of the restructuring of neural networks, and the presence of neuronogenesis throughout the entire postnatal life of the mammalian organism.
Sources of neural stem cells
Human neural stem cells are isolated during operations on the subventricular region of the lateral ventricles and the dentate gyrus of the hippocampus, whose cells form neurons in the culture (neural spheres), and after dispersing and preforming the latter, all major cell types of the central nervous system or, in a special medium, new microspheres. In suspension cultures of dissociated tissue, isolated from the periventricular parts of the embryonic brain, neurospheres also appear.
The markers of immature brain cells are nestin, beta-tubulin III (marker of the neuronal line), vimentin, GFAP and NCAM, for the immunocytochemical identification of which monoclonal antibodies are used. Nestin (a protein of intermediate type IV neurofilaments) expresses multipotent neuroectodermal cells. This protein is used to identify and isolate multipotent neuroepithelial progenitor cells from the CNS using Rat-401 monoclonal antibodies that can detect up to 95% of neural tube cells in rat embryos on the eleventh day of gestation. Nestin is not expressed on the differentiated descendants of neural stem cells, but is present in early neural progenitor cells, postmitotic neurons, and early neuroblasts. With the help of this marker, neuroepithelial progenitor cells have been identified and the existence of stem cells in the central nervous system has been proved. Vimentin (a protein of intermediate type III neurofilaments) is expressed by neural and glial progenitor cells, as well as by neurons, fibroblasts and smooth muscle cells. Consequently, both immunocytochemical markers do not have the specificity necessary for the separate identification of neural stem and progenitor cells. By using beta-tubulin III, the neuronal orientation of stem cell differentiation is established, whereas type I astrocytes are identified by GFAP expression, and oligodendrocytes specifically express galactocerebroside (Ga! C).
Mitogens for neural progenitor cells are FGF2 and EGF, which support the proliferation of undifferentiated progenitor cells in culture with the formation of neurospheres. The rate of division of neural stem cells increases significantly under the influence of FGF2, and also when using the combination FGF2 + EGF. The proliferative effects of FGF2 are mediated by FGF2-R1 receptors. Heparin increases the affinity of the receptor binding of FGF2 and dramatically enhances its mitogenic effect on neuroepithelial cells. In the early stages of embryogenesis, FGF2 receptors are expressed in the rat telencephalon, at later stages their localization is limited to the ventricular zone. Peak expression of FGF2-R1 by postmitotic cells is observed after the end of the period of early neurogenesis. The initial period of development of telencephalon is characterized by a low level of expression of EGF receptors, mainly in the cells of the ventral region. In the later stages of embryogenesis, EGF-R expression rises in the dorsal direction. In the brains of rodents, EGF has a high affinity for the transforming growth factor beta (TGF-beta-R) receptor, with which it binds predominantly. Indirectly, the functional role of EGF-R is evidenced by data on cortical dysgenesis of the forebrain arising during late embryogenesis and postnatal ontogenesis, decreased forebrain function, death of cortical cells and ectopia of the hippocampus in mice with knockout of the EGF receptor gene. In addition, the presence of TGF-a in the nutrient medium is absolutely essential for the formation of the neurosphere. After the removal of growth factors from the conditioned medium, the cells cease to divide and undergo spontaneous differentiation with the formation of neurons, astrocytes and oligodendroblasts.
Considering this, the reaggregation of dissociated stem cells and the cultivation of neurospheres are carried out in nutrient media containing EGF and basic FGF or FGF2, but without the addition of serum. It is shown that EGF induces proliferation of stem cells in the subendendic zone of the lateral ventricles, and the main FGF promotes the proliferation of stem cells of the striatum, hippocampus, new cortex and optic nerve of the mature brain. The combination of EGF and basic FGF is absolutely necessary for the active proliferation of stem cells isolated from the ependyma of the third and fourth ventricles of the forebrain, as well as from the spinal canal of the thoracic and lumbar spinal cord.
After dissociation, a suspension of neural stem cells is cultured in plastic dishes or in multi-well plates without an adhesive substrate to increase the size of the emerging new neurospheres, which usually takes about 3 weeks. The method of multiple dispersion and reproduction of neurospheres allows obtaining a sufficient number of linear clones of multipotent stem cells for intracerebral transplantation. This principle is also based on the creation of a bank of stem cells isolated from the human embryonic brain. Their long (for several years) cloning makes it possible to obtain stable lines of neural stem cells, from which catecholaminergic neurons are formed during induced differentiation.
If neurospheres are not dispersed and grown on adhesive substrates in media devoid of growth factors, proliferating stem cells begin to spontaneously differentiate to form neuronal and glial progenitor cells with the expression of markers of all types of nerve cells: MAP2, Tau-1, NSE, NeuN, beta -tubulin III (neurons), GFAP (astrocytes), and CalC, 04 (oligodendrocytes). Unlike mouse and rat cells, in human neural stem cell cultures, neurons account for more than 40% of all differentiated cells (1 to 5% in rodents), but much less oligodendrocytes occur, which is very important from the point of view of cell therapy for demyelinating diseases. The problem is solved by adding a B104 culture medium, which stimulates the formation of myelin-producing cells.
In the cultivation of neural progenitor cells in the brain of human embryos in a medium containing EGF, basic FGF and LIF, the number of progenitor cells of the neural line is increased by 10 million fold. Reproduced in vitro cells retain the ability to migrate and differentiate into nerve and glial cells after transplantation into the brain of sexually mature rats. However, in vivo, the number of divisions of multipotent progenitor cells is limited. It has been repeatedly noted that the Hayflick limit for the "adult" neural stem cell (about 50 mitoses) is not yet achievable even in the experiment - cells in the form of neurospheres retain their properties only for 7 months and only at 8 passages. It is believed that this is due to the peculiarities of their dispersion during passaging (trypsinization or mechanical action), which drastically reduces the proliferative activity of cells due to the violation of intercellular contacts. Indeed, if instead of dispersing a method of dividing the neurospheres into 4 parts is used, the viability of the cells during passage is significantly increased. This technique allows the cultivation of human neural stem cells for 300 days. However, after this period, the cells lose mitotic activity and are degenerated or go to the stage of spontaneous differentiation with the formation of neurons and astrocytes. On this basis, the author considers that 30 mitoses is the limiting number of divisions for cultured neural stem cells.
When culturing human neural stem cells in vitro, mainly GABA -ergic neurons are formed. Without creating special conditions, neural progenitor cells give rise to dopaminergic neurons (necessary for cell therapy of Parkinson's disease) only in the first passages, after which all neurons in culture consist exclusively of GABA-ergic cells. In rodents, the induction of dopaminergic neurons in vitro is caused by IL-1 and IL-11, as well as fragments of nerve cell membranes, LIF and GDNF. However, this method was unsuccessful for a man. Nevertheless, with intracerebral GABA-ergic neuronal transplantation in vivo under the influence of microenvironment factors, nerve cells with different mediator phenotypes appear.
The search for combinations of neurotrophic factors has shown that FGF2 and IL-1 induce the formation of dopaminergic neuroblasts, which, however, are not capable of producing dopaminergic neurons. The differentiation of stem cells of the hippocampus into stimulating glutamatergic and inhibitory GABAergic neurons occurs under the influence of neurotrophins, and EGF and IGF1 induce the formation of glutamatergic and GABAergic neurons from neural precursor cells of human embryos. The sequential addition of retinoic acid and neurotrophin 3 (NT3) to the culture significantly increases the differentiation of stem cells of the hippocampus of the mature brain into neurons of different mediator nature, whereas a combination of the neurotrophic brain factor (BNDF), NT3 and GDNF in cultures of the hippocampus and the new cortex pyramidal neurons.
Thus, the results of numerous studies indicate that, firstly, stem cells from different brain structures under the influence of local specific tissue factors are able to differentiate in vivo into the neuronal phenotypes inherent in these structures. Secondly, the directed induced differentiation of neural stem cells in vitro by cloning progenitor cells allows the production of nerve and glial cells with predetermined phenotypic characteristics for intracerebral transplantation in various forms of brain pathology.
There is no doubt that pluripotent stem cells isolated from embryos or the CNS of an adult can be considered as a source of new neurons and used in a clinic for the purpose of treating neurological pathology. However, the main obstacle to the development of practical cellular neurotransplantation is the fact that most neural stem cells do not differentiate into neurons after implantation into non-neurogenic areas of the mature CNS. Bypassing this obstacle, a very original innovative method is proposed that allows in vitro to obtain a pure population of neurons from human fetal neural stem cells after transplantation into the CNS of a mature rat. The authors prove that the differentiation of cells implanted according to this method results in the formation of neurons of the cholinergic phenotype, which is caused by the influence of environmental microenvironment factors. The proposed technology is of interest from the point of view of developing new types of therapy based on stem cells and replacing damaged neurons due to trauma or neurodegenerative disease, since cholinergic neurons play a leading role in the formation of motor functions, memory functions and training. In particular, cholinergic neurons isolated from human stem cells can be used to replace motoneurons lost in amyotrophic lateral sclerosis or spinal cord injuries. At the present time, there is no information on the methods of producing a significant number of cholinergic neurons from a population of stem cells preformed by mitogen. The authors propose a fairly simple but effective method of stimulating mitogen preformed primary human neural stem cells in the direction of development into practically pure neurons after implantation both in non-neurogenic and in the neurogenic zones of the sexually mature rat. The most important result of their work is the transformation of a sufficiently large number of transplanted cells into cholinergic neurons when implanted into the middle membrane and spinal cord.
In addition, various combinations of the following trophic factors and chemical elements are proposed for the preformation of the neuronal stem cells of the cerebral cortex of the 8-week human embryo into cholierergic neurons in vitro: recombinant basic FGF, EGF, LIF, amino-terminal murine sound peptide (Shh-N ), transretinic acid, NGF, BDNF, NT3, NT4, natural laminin and mouse heparin. The original line of human neural stem cells (K048) was maintained in vitro for two years and sustained 85 passages without changes in proliferative and differentiating properties with the preservation of a normal diploid karyotype. The non-dispersed neurotransfers of the 19-55th passages (38-52 weeks) were planted on poly-d-lysine and laminin, and then treated with the above-mentioned factors in varying concentrations, combinations, and sequences. The combination, consisting of the basic FGF, heparin and laminin (in the abbreviation FHL), had a unique effect. After one day of culturing the neural stem cells of the embryo in the FHL medium with or without Shh-N (combination of Shh-N + FHL in the abbreviation SFHL), rapid multiplication of large planar cells was observed. All other one-day protocols (for example, such as the basic FGF + laminin), on the contrary, led to limited radial proliferation of spindle-shaped cells, and these cells did not leave the core of the neurosphere. After 6 days of activation and subsequent ten-day differentiation in a medium containing B27, large polypolar neuron-like cells were found at the edge of the FHL-activated spheres. In other protocol groups, most neuron-like cells remained small and bipolar or unipolar. Immunocytochemical analysis showed that small (<20 μm) bipolar or unipolar cells were either GABA -ergic or glutamatergic, whereas most large polypolar cells localized at the edge of FHL-activated neurospheres turned out to be cholinergic, as they expressed the markers characteristic of cholinergic neurons (Islet-1 and ChAT). Some of these neurons simultaneously expressed synapse 1. As a result of five series of independent experiments, the authors found that the total population of cells in single-layer zones was differentiated by 45.5% in neurons TuJl +, while cholinergic (ChAT ^) neurons were only 27.8 % of cells in the same population. After 10 days of additional in vitro differentiation, in addition to the cholinergic neurons, a significant number of small neurons-glutamatergic (6.3%), GABA-ergic (11.3%), and astrocytes (35.2% ) and nestinpositive cells (18.9%). When using other combinations of growth factors, cholinergic neurons were absent, and the edge cells of the neurosphere were formed either by astrocytes or by small glutamatergic and GABA -ergic neurons. Monitoring of standby and active potentials using the whole-cell patch clamp technique showed that after seven days of FHL activation, most of the large polipolar cells had a rest potential of -29.0 ± 2.0 mV, in the absence of the action potential. After 2 weeks, the resting potential increased to -63.6 ± 3.0 mV, and action potentials were observed at the time of induction of depolarizing currents and blocked with 1M tetrodotoxin, which indicates the functional activity of cholinergic immature neurons.
Further, the authors found that FHL or SFHL activation in vitro alone does not lead to the formation of mature neurons, and attempted to establish whether stem cells prefigured with FHL or SFHL can differentiate into cholinergic neurons when transplanted into the CNS of a mature rat. For this purpose, the activated cells were injected into the neurogenic zone (hippocampus) and into several non-neurogenic zones, including the prefrontal cortex, the middle membrane and the spinal cord of adult rats. Tracking of the implanted cells was carried out with the help of the CAO - ^ p vector. It is known that OCD marks simultaneously both the ultrastructure of the cell and the cellular processes (molecular level) without leakage and can be directly visualized. In addition, OPP-labeled neural stem cells maintain a profile of neuronal and glial differentiation, identical to the profile of unconverted stem cells of the embryonic brain.
One to two weeks after the implantation of 5 x 10 4 activated and labeled neural stem cells, they were found in the spinal cord or brain of rats, with OCD + cells mostly located near the injection site. The processes of migration and integration were observed already a month after transplantation. The limits of migration varied depending on the injection site: when injected into the prefrontal cortex the OCD + cells were located 0.4-2 mm from the zone of administration, in the case of implantation in the middle membrane, hippocampus or spinal cord, the cells migrated to much larger distances up to 1-2 cm. Transplanted cells were localized in highly organized structures of the central nervous system, including the frontal cortex, the middle membrane, the hippocampus and the spinal cord. OCD-tagged neuronal elements were seen already at the first week after transplantation, and their number increased significantly 1 month after the operation. Stereological analysis showed a higher survival rate of implanted cells in different structures of the brain, in comparison with the dorsal.
It is known that in most tissues of an adult mammalian organism the population of regional stem cells is preserved, the transformation of which into mature cells is regulated by specific tissue factors. The proliferation of stem cells, the differentiation of progenitor cells and the formation of neuronal phenotypes specific for this brain structure in vivo are much more pronounced in the embryonic brain, which is determined by the presence of high concentrations of morphogenetic factors of the local microenvironment - neurotrophins BDNF, NGF, NT3, NT4 / 5 and growth factors FGF2, TGF-a, IGF1, GNDF, PDGF.
Where are the neural stem cells?
It is established that neural stem cells express glial acid fibrillar protein, which among mature cells of the neural line is preserved only on astrocytes. Therefore, the stem reserve in the mature central nervous system may be astrocytic cells. Indeed, neurons derived from GFAP-positive progenitors were detected in the olfactory bulbs and dentate gyrus, which contradicts the traditional notions of the progenitor role of radial glia that does not express GFAP in the dentate gyrus at adulthood. It is possible that in the central nervous system there are two populations of stem cells.
The question of the localization of stem cells in the subventricular zone also remains unclear. According to some authors, ependymal cells form spherical clones in culture, which are not true neurospheres (as clones of subendendic cells), since they are able to differentiate only in astrocytes. On the other hand, after the fluorescent or viral labeling of ependyma cells, a marker is found in the cells of the subendum layer and the olfactory bulbs. Such labeled cells in vitro form neurospheres and differentiate into neurons, astrocytes and oligodendrocytes. In addition, it is shown that in the ependymium about 5% of the cells express stem markers - neustin, Notch-1 and Mussashi-1. It is assumed that the mechanism of asymmetric mitosis is associated with a non-uniform distribution of the membrane receptor Notch-1, as a result of which the latter remains on the membrane of the daughter cell located in the ependymal zone, whereas the parent cell migrating to the subendendic layer is deprived of this receptor. From this point of view, the subependymic zone can be considered as a collector of progenitor precursors of neurons and glia formed from the stem cells of the ependymal layer. In the opinion of other authors, in the caudal divisions of the subventricular zone only glial cells are formed, and the cells of the rostral-lateral region are the source of the neuronogenesis. In the third variant, the anterior and posterior parts of the subventricular zone of the lateral ventricles are given equivalent neurogenic potencies.
The fourth variant of the organization of the stem reserve in the central nervous system is preferable, according to which three main types of neuronal progenitor cells - A, B and C - are isolated in the subventricular zone. The A-cells express the early neuronal markers (PSA-NCAM, TuJl) and are surrounded by B cells, which are identified by the expression of antigens as astrocytes. C-cells, not having antigenic characteristics of neurons or glia, have high proliferative activity. The author has convincingly proved that B cells are precursors of A-cells and de novo-forming neurons of olfactory bulbs. During migration, A-cells are surrounded by strands of neural progenitor cells, which differs significantly from the migration mechanism of postmitotic neuroblasts along radial glia in the embryonic brain. Migration is completed in the olfactory bulbs by mitotic division of both A- and B-cells, the derivatives of which are built into the layers of granular cells and into the glomerular layer of the olfactory zone of the brain.
In the developing brain of embryos, there are no differentiated ependymal cells, and ventricular walls include multiplying stem cells of the ventricular hermetic and subventricular zones, where primary neuro- and glioblastes migrate. On this basis, some authors believe that the subependymic region of the mature brain contains a reduced embryonic hermetic nerve tissue, consisting of astrocytes, neuroblasts, and unidentified cells. True neural stem cells account for less than 1% of cells in the hermetic zone of the lateral ventricular wall. Partly, therefore, and also in connection with the evidence that astrocytes of the subendum zone are the precursors of neural stem cells, the possibility of transdifferentiation of astrocytic glial elements with the acquisition of neuronal phenotypic characteristics is not ruled out.
The main obstacle to the final solution of the problem of the localization of neural stem cells in vivo is the absence of specific markers for these cells. Nevertheless, from a practical point of view, it seems very interesting that neural stem cells were isolated from the central nervous system, which do not contain subependymic zones - the third and fourth ventricles of the forebrain, the spinal canal of the thoracic and lumbar spinal cord. Especially important is the fact that with damage to the spinal cord, the proliferation of stem cells of the ependyma of the central canal is enhanced, with the formation of progenitor cells migrating and differentiating into gliomesodermal scar astrocytes. In addition, the precursor cells of astro- and oligodendrocytes are also found in the intact spinal cord of adult rats.
Thus, the literature data convincingly testify to the presence in the central nervous system of adult mammals, including humans, a regional stem reserve, whose regenerative-plastic capacity, unfortunately, is able to provide only physiological regeneration processes with the formation of new neuronal networks, but does not correspond to the needs of reparative regeneration. This raises the problem of finding ways to increase the CNS stem resources exogenously, which is insoluble without a clear idea of the mechanisms of CNS formation in the embryonic period.
Today we know that in the process of embryonic development stem cells of the neural tube are the source of cells of three types - neurons, astrocytes and oligodendrocytes, that is, neurons and neuroglia come from a single precursor cell. The differentiation of the ectoderm into clusters of neural progenitor cells begins under the influence of proineural gene products of the bHLH family and is blocked by the expression of receptor transmembrane protein derivatives of the Notch family that limit the determination and early differentiation of neural progenitor cells. In turn, Notch receptor ligands are the transmembrane proteins Delta of neighboring cells, due to the extracellular domain of which direct intercellular contacts are made with inductive interaction between stem cells.
Further implementation of the program of embryonic neurogenesis is no less complex and, it would seem, should be species-specific. However, the results of neuroxenotransplantation studies indicate that stem cells have a pronounced evolutionary conservatism, so that human neural stem cells are able to migrate and develop when they are transplanted into the brain of a rat.
It is known that the mammalian CNS has an extremely low ability for reparative regeneration, which is characterized by the absence of any signs of the emergence of new cellular elements in the mature brain in return for the neurons that died as a result of trauma. However, in the case of neuroblast transplantation, the latter not only survive, proliferate and differentiate, but are also capable of being integrated into brain structures and functionally replacing lost neurons. When the committed neuronal progenitor cells were transplanted, the therapeutic effect was significantly weaker. Such cells showed a low capacity for migration. In addition, neural progenitor cells do not reproduce the architecture of neural networks and functionally do not integrate into the brain of the recipient. In connection with this, the issues of reparative-plastic regeneration are actively studied in the transplantation of unformed multipotent neural stem cells.
In the study of M. Aleksandrova and co-authors (2001), in the first variant of the experiments, mature female rats acted as recipients, and embryos of 15-day development were donors. Recipients were removed a portion of the occipital cortex of the brain and a mechanically suspended tissue of the presumptive embryonic cortex was transplanted into the cavity, containing multipotent stem cells of the ventricular and subventricular regions. In the second variant of the experiments, the neural stem cells of the 9-week human embryo were transplanted into the brain of the sexually mature rats. From the periventricular region of the brain of embryos, the authors isolated pieces of tissue, placed them in the F-12 nutrient medium and, by repeated pipetting, received a cell suspension, and then cultured them in a special NPBM medium supplemented with growth factors - FGF, EGF and NGF. Cells were grown in a suspension culture prior to the formation of neurospheres, which were dispersed and again planted in the culture. After 4 passages with a total cultivation period of 12-16 days, the cells were used for transplantation. The recipients were 10-year-old rat rats and mature two-month-old Wistar rats, who received 4 μl of a suspension of human neural stem cells to the region of the lateral ventricle of the brain without immunosuppression. The results of the work showed that the dissociated cells of the ventricular and subventricular embryonic cortex of the cerebral cortex during allotransplantation into the mature brain continued their development, that is, the factors of the microenvironment of the differentiated brain of the recipient did not block the growth and differentiation of the neural stem cells of the embryo. In the early periods after transplantation, multipotent cells continued to mitotic division and actively migrated from the transplantation area to the brain tissue of the recipient. Transplanted embryonic cells with a huge migration potential were found in virtually all layers of the cortex of the recipient's brain along the transplantation track and in white matter. The length of the migration tract of nerve cells has always been much smaller (up to 680 microns) than glial elements (up to 3 mm). Structural vectors for the migration of astrocytes were blood vessels and fibrous structures of the brain, which was noted in other studies.
Earlier it was believed that the accumulation of labeled astrocytes in the area of damage to the cerebral cortex of the recipient may be due to the formation of a glial barrier between the tissues of the graft and the recipient. However, a study of the structure of compactly located cellular grafts showed that their cytoarchitectonics is characterized by randomness, without any layered distribution of transplanted cells. The degree of ordering of the transplanted neurons approximated that of cells of the normal cerebral cortex only if there is no glial barrier between the donor and recipient tissues. Otherwise, the structure of the cells of the transplant was atypical, and the neurons themselves underwent hypertrophy. Neuroimmunochemical typing of transplanted cells in transplants revealed inhibitory GABA -ergic neurons to reveal the expression of PARV, CALB and NPY proteins. Consequently, in the mature brain, microenvironment factors that can support proliferation, migration, and specific differentiation of neural multipotent cells persist.
In the culture of human stem cells isolated from the periventricular region of the brain of 9-week-old embryos, M. Alexandrova and coauthors (2001) found a large number of non-stingpositive multipotent cells in the fourth passage, some of which had already undergone in vitro differentiation and developed according to the neuronal type, results of research by other authors. After transplantation into the brain of adult rats, cultured human stem cells were mitotically divided and migrated into the tissue of the xenogen brain of the recipient. In cell transplants, the authors observed two populations of cells - small and larger. The latter migrated both in the parenchyma and in the fiber structures of the recipient brain for insignificant distances - within 300 μm. The greatest extent of the migration path (up to 3 mm) was characteristic of small cells, some of which were differentiated into astrocytes, which was established using monoclonal antibodies to GFAP. Both types of cells were found in the lateral ventricular wall, which indicated the release of transplanted cells to the rostral migration tract. The astrocyte derivatives of neural stem cells, both human and rats, migrated predominantly through the blood capillaries and the fiber structures of the recipient's brain, which coincides with the data of other authors.
Analysis of the differentiation of human stem cells in vivo using monoclonal antibodies to GFAP, CALB and VIM revealed the formation of both astrocytes and neurons. Unlike the cells of rat grafts, many human stem cells were vimentin-positive. Consequently, part of the human multipotent cells was not differentiated. Later the same authors showed that human neural stem cells transplanted without the use of immunosuppression survive after transplantation in the rat brain for 20 days in the absence of signs of immune aggression from the glial elements of the mature brain.
It has been established that even the Drosophila neural stem cells survive and undergo differentiation in the brain of a taxon as far away from insects as a rat. The correctness of the experiment of the authors is not in doubt: the transgenic lines of Drosophila contained the genes of neurotrophic human factors NGF, GDNF, BDNF, inserted into the CaSper vector under the Drosophilic hit-shock promoter, so that the mammalian body temperature automatically caused their expression. The authors identified Drosophila cells from the bacterial galactosidase gene product by histochemical X-Gal staining. In addition, it turned out that the Drosophila neural stem cells specifically react to neurotrophic factors encoded by human genes: when xenotransplantation of cells of the transgenic Drosophila line containing the gdnf gene, the tyrosine hydroxylase synthesis was dramatically increased in its differentiating stem nerve cells, and the cells with the ngf gene actively produced acetylcholinesterase . Similar gene-dependent xenograft reactions induced in the embryonic neural tissue allograft transplanted with it.
Does this mean that the specific differentiation of neural stem cells is induced by vidon-specific neurotrophic factors? According to the results of the authors, a xenograft that produces neurotrophic factors had a specific effect on the fate of allografts, which in this case developed more intensively and 2-3 times larger than allografts introduced into the brain without the addition of xenografts. Consequently, xenograft cells containing the genes of neurotrophins, in particular the gene encoding the glial neurotrophic factor (GDNF) of a human, exert a vidon-specific effect on the development of the allograft, similar to that of the corresponding neurotrophin. It is known that GDNF increases the survival of dopaminergic neurons in the embryonic midbrain of rats and enhances the metabolism of dopamine by these cells, and also induces the differentiation of tyrosine hydroxylase-positive cells, enhancing the growth of axons and increasing the body size of neurons. Similar effects are observed in the culture of dopaminergic neurons in the rat mid-brain.
After xenotransplantation of human neural stem cells into the brain of mature rats, their active migration is noted. It is known that the process of migration and differentiation of neural stem cells is controlled by a set of special genes. The initiating migratory signal to the progenitor cell at the onset of differentiation yields the protein product of the proto-oncogene c-ret together with GDNF. The next signal comes from the gene mash-1, which controls the choice of the path of cell development. In addition, the specific reaction of differentiating cells also depends on the a-receptor of the ciliary neurotrophic factor. Thus, taking into account the completely different genetic constitution of the xenogeneic human neural stem cells and the brain cells of the recipient-rat, it is necessary to recognize not only the species-specific nature of neurotrophic factors, but also the highest evolutionary conservatism of the genes responsible for the specific differentiation of neural stem elements.
Whether the xenotransplantation of embryonic neuromaterial in neurosurgical practice of treatment of neurodegenerative pathological processes, caused by violation of myelin synthesis by oligodendrocytes, will be possible, the future will show. In the meantime, the most intensively resolving issues of neurotransplantation associated with obtaining from the embryonic or mature brain allogenic neural stem cells in the culture and their subsequent directed differentiation into neuroblasts or specialized neurons.
Transplantation of neural stem cells
To stimulate proliferation and differentiation of the neural stem cells of an adult organism, it is possible to transplant the embryonic nerve tissue. It is possible that the stem cells of the embryonic nervous tissue that are introduced with the allograft can themselves undergo proliferation and differentiation. It is known that after spinal injury regeneration of nerve conductors is carried out by elongation of damaged axons and collateral scotting of axons of undamaged motor neuron outgrowths. The main factors impeding the regeneration of the spinal cord are the formation of connective tissue scar in the area of damage, degenerative and degenerative changes in central neurons, NGF deficiency, and the presence of myelin degradation products in the affected area. It has been shown that transplantation into the damaged spinal cord of different types of cells - sciatic nerve fragments of adult animals, embryonic occipital cortex, hippocampus, spinal cord, Schwann cells, astrocytes, microglia, macrophages, fibroblasts - promotes the regeneration of damaged axons by sprating and allows newly formed axons to germinate area of spinal cord injury. It has been experimentally proved that transplantation of embryonic neural tissue into the area of spinal cord injury through the action of neurotrophic factors accelerates the growth of affected axons, prevents the formation of glial scar and the development of degenerative and degenerative processes in central neurons, while cells of transplanted embryonic neural tissue survive in the spinal cord, integrate with adjacent tissues and promote the germination of axons through the affected area with the formation of synapses of dens of the drtic type on spinal neurons.
This direction of regenerative and plastic medicine has received the greatest development in Ukraine due to the work of the scientific team under the leadership of V.I. Tsymbalyuk. First of all, these are experimental studies of the efficiency of transplantation of embryonic neural tissue in spinal cord injuries. In the autotransplantation of the peripheral nerve, the authors observed the most pronounced changes in the destructive character in the zone of the distal suture, where they were combined with reparative processes on the 30th day after the operation. At allotransplantation the morphofunctional state of the implanted nerve on the 30th day was characterized by severe destruction with the phenomena of fatty degeneration and amyloidosis against the background of focal inflammatory lymphoid cell infiltration with the predominant atrophy of Schwann cells. Transplantation of the embryonic nerve tissue to a greater extent contributed to the restoration of conduction of the spinal cord, especially in animals that were operated during the first 24 hours after the trauma: against a background of a decrease in the intensity of inflammatory-destructive processes, hypertrophy and hyperplasia of protein-synthesizing and energy-producing ultrastructural elements of spinal neurons, hypertrophy and hyperplasia of oligodendrocytes, the amplitude of the muscle action potential was restored by 50% and by 90% the rate holding momentum. When assessing the effectiveness of transplantation of embryonic neural tissue, depending on the transplant zone, it was found that the best results are observed when the transplant is inserted directly into the zone of spinal cord injury. With the complete intersection of the spinal cord, transplantation of embryonic neural tissue was ineffective. Dynamic studies have shown that the optimal time for performing embryonic nerve tissue transplantation is the first 24 hours after a spinal cord injury, whereas an operation in the period of pronounced secondary ischemic-inflammatory changes occurring on the 2-9th day after trauma should be considered inexpedient.
It is known that severe craniocerebral trauma provokes a powerful and long-lasting activation of lipid peroxidation at the initial and intermediate stages of the post-traumatic period both in damaged brain tissue and in the body as a whole, and it also disrupts the processes of energy metabolism in the injured brain. Under these conditions, transplantation of embryonic neural tissue into the area of traumatic injury promotes stabilization of lipid peroxidation processes and increases the potential of the antioxidant system of the brain and the organism as a whole, enhances its antiradical protection on the 35th-60th day of the post-traumatic period. At the same time after transplantation of embryonic nerve tissue, energy metabolism and oxidative phosphorylation in the brain are normalized. In addition, it is shown that on the first day after the experimental craniocerebral trauma, the impedance of the tissue of the injured hemisphere decreases by 30-37%, the contralateral one by 20%, which indicates the development of generalized cerebral edema. In animals that underwent transplantation of embryonic nerve tissue, the edema of the edema occurred much faster - on the seventh day the mean value of the impedance of the tissues of the injured hemisphere reached 97.8% of the control level. Moreover, the complete restoration of the impedance values on the 30th day was noted only in animals to which the embryonic nerve tissue was transplanted.
The death of a part of brain neurons after a severe craniocerebral injury is one of the main causes of post-traumatic complications. The neurons of integrating dopaminergic and noradrenergic systems of the middle and oblong brain are especially sensitive to trauma. A decrease in the level of dopamine in the striopallidal complex and the cerebral cortex significantly increases the risk of development of motor disorders and mental disorders, epileptiform states, and a decrease in dopamine production in the hypothalamus can cause numerous vegetative and somatic disorders observed in the distant post-traumatic period. The results of the studies carried out in the experimental craniocerebral trauma indicate that transplantation of embryonic neural tissue contributes to the restoration of dopamine in the injured hemisphere of the brain, dopamine and norepinephrine in the hypothalamus, as well as to increased levels of norepinephrine and dopamine in the median and oblong brain. In addition, as a result of transplantation of embryonic nerve tissue in the injured hemisphere of the brain of experimental animals, the percentage of phospholipids normalizes and the content of fatty acids increases (C16: 0, C17: 0, C17: 1, C18: 0, C18: 1 + C18: 2, C20 : 3 + C20: 4, C20: 5).
These data confirm the stimulation of regenerative-plastic processes by transplanted embryonic neural tissue and indicate a reparative-trophic effect of the graft on the recipient's brain as a whole.
Special attention should be paid to the clinical experience of the staff of the Institute of Neurosurgery. A.P. Romodanova AMS of Ukraine on transplantation of embryonic nerve tissue in children's cerebral palsy - an extremely complex pathology with gross violations of motor function. Clinical forms of infantile cerebral palsy depend on the level of damage to the integral structures responsible for the regulation of muscle tone and the formation of motor stereotypes. Currently, there is sufficient evidence in favor of the fact that in the violations of motor functions and muscle tone an important role is played by pathological changes in the system of striopalloid-thalamocortical motor control. The striospallidal link of this system exercises a controlling function through the nigrostri- nary production of dopamine. The direct route to the realization of thalamocortical control begins from neurons of the shell, is mediated by gammaaminobutyric acid (GABA) and substance P and is projected directly into the motor zone of the inner segment of the pale sphere and the black substance. An indirect pathway, the effect of which is realized with the participation of GABA and enkephalin, originates from the neurons of the shell and affects the nuclei of the basal ganglia through a sequence of compounds that include the outer segment of the pale sphere and the subthalamic nucleus. Disturbances in the conductivity of the direct path cause hypokinesia, whereas a decrease in the conductivity of the structures of the indirect path leads to hyperkinesia with corresponding changes in the muscle tone. The integrity of GABA-ergic conductive paths at different levels in the motor control system and the integration of dopaminergic bonds at the shell level are essential for the regulation of thalamocortical interactions. The most common manifestation of motor pathology in various forms of infantile cerebral palsy is a violation of muscle tone and a closely related change in the reflex activity of the muscles.
Transplantation of embryonic neural tissue in children's cerebral palsy requires a careful analysis of the nature of damage to the brain structures. Based on the determination of the content of dopamine and GABA in the subarachnoid liquor, the authors detailed the level of disruption of the integration of the functional structures of the brain, which made it possible to objectify the results of the operative intervention and to correct the repeated neurotransplantations. Embryonic nerve tissue (abortion material of the 9-week-old embryo) was transplanted into the parenchyma of the cortex of the precentral gyrus of the cerebral hemispheres, depending on the severity of the atrophic changes. In the postoperative period, no complications or deterioration of the patients were observed. Positive dynamics was noted in 63% of patients with spastic forms, in 82% of children with an atonic-aesthetic form and only in 24% of patients with a mixed form of the disease. A negative effect on the results of the operation of a high level of neurosensitivity with the presence of autoantibodies to neurospecific proteins was established. Inadequate transplantation of embryonic neural tissue was found in patients aged 8-10 years and older, as well as in severe hyperkinetic syndrome and episyndrome. Clinically, the effectiveness of transplantation of embryonic nerve tissue in patients with spastic forms of infantile cerebral palsy manifested itself in the formation of new statomotor skills and voluntary movements with correction of the pathological motor pattern and a reduction in the degree of spasticity, pathological poses and attitudes. The authors believe that the positive effect of transplantation of embryonic nerve tissue is the result of a normalizing effect on the functional activity of the supraspinal structures involved in the regulation of the tone of postures and voluntary movements. In this case, the positive clinical effects of transplantation of embryonic neural tissue are accompanied by a decrease in the content of neurotransmitters in the subarachnoidal CSF, which indicates the restoration of integral interactions of affected brain structures.
There is one more severe form of neurological pathology - the apallic syndrome, the problem of whose treatment, unfortunately, is far from being solved. Apallic syndrome is a polyethological subacute or chronic condition resulting from severe organic CNS lesions (mainly the cerebral cortex) and is characterized by the development of panapraxia and panognosia with relatively preserved function of segmental-stem divisions and the formation of the limbic-reticular brain complex. Follow-up studies (from 1 to 3 years) showed that the apallic syndrome is not the final diagnosis of persistent neurodevelopment in children, but is transformed either into organic dementia or into a chronic vegetative state. In the Department of Rehabilitation Neurosurgery of the Institute of Neurosurgery. A.P. Romodanova AMS Ukraine 21 patients with the effects of apallic syndrome performed transplantation of embryonic nerve tissue. Under the general anesthesia, a milling hole was placed over the zone of the most pronounced atrophic changes revealed in computer or magnetic resonance imaging, and in the presence of diffuse atrophy of gray or white matter, the graft was inserted into the precentral and central gyrus of the brain. After opening the dura mater, the pieces of tissue from the bookmark of the sensorimotor cortex of 8-9 week old embryos were implanted intracortically using a special device. The number of samples of the implanted tissue was 4 to 10, which was determined by the size of the milling hole and the size of the local changes in the medulla. Unlike other types of pathology, with the apallic syndrome, the authors sought to implant as much embryonic tissue as possible in the most accessible areas of the brain. The dura mater was sutured, the plastic of the skull defect was made. During the operation, all the patients showed marked changes both from the side of the cortex (atrophy, absence of convolutions, discoloration and pulsation of the brain substance), and the membranes of the brain (thickening of the dura mater, considerable thickening of the arachnoid shell with the presence of its own blood vessels in it, fusion shells with the underlying brain substance). These changes were more pronounced in patients, in whose anamnesis there were indications on the transferred inflammatory defeats of the brain. In patients who underwent hypoxia of the central nervous system, diffuse atrophic changes in the medulla, especially the cortical areas, predominated with an increase in the subarachnoid space, without pronounced changes from the cerebral membranes. Half of the patients showed increased bleeding of soft tissues, bones, brain substance. After operations in the period from six months to three years, the condition improved in 16 patients, five patients remained unchanged. Positive dynamics was observed both from the side of the motor and mental sphere. Muscular tone decreased in ten patients, and the motor activity increased in patients (paresis decreased, coordination of movements improved), and in five children the manipulative ability of the upper limbs increased markedly. In four patients, the incidence and severity of epileptic seizures decreased, and one child had no seizures during the entire follow-up period after the operation. Aggressiveness decreased in two children, in two patients with severe bulbar disorders, the swallowing act improved, two children could chew themselves 2 weeks after the operation. There was a decrease in the severity of mental disorders, nine children after the operation became calmer, sleep and attention improved in seven patients. Three patients with the effects of the aphallic syndrome began to recognize their parents, one - to follow instructions, two - to pronounce words, the three of them decreased the degree of dysarthria. The authors note that a noticeable improvement in the condition of patients begins 2 months after the operation, reaches a maximum by 5-6 months, then the rate of improvement slows down and by the end of the year in 50% of the patients the process stabilizes. The positive effect of neurotransplantation served as the basis for re-operation in six patients with the effects of the apallic syndrome, but in the other hemisphere of the brain. The technique and technique of the second transplantation were identical to those in the first operation, but the clinical effect of the second operation was lower, although no serious complications occurred after the first and after the second operative intervention. According to the authors, the mechanism of the therapeutic effect of neurotransplantation is associated with the neurotrophic effect of transplanted embryonic nerve tissue, which contains a large number of growth, hormonal and other biologically active substances that stimulate the repair of damaged neurons and plastic reorganization of the brain tissue of the recipient. It is not excluded and activating influence on the activity of nerve cells, which previously were morphologically preserved, but because of the disease they lost their functional activity. It is the rapid neurotrophic action that can explain the improvement of bulbar functions in some children at the end of the first and second weeks after the operation. It is assumed that along with this, by the third or fourth month between the transplant and the brain of the host morphofunctional bonds are established, through which the neurotransplant replaces the function of dead brain cells, which is the substrate for improving both motor and mental functions of patients.
The effect of the graft of the embryonic neural tissue on the reorganization of inter-neural connections was studied experimentally. Authors on white rats with the help of fluorescent lipophilic label DIL (1,1-dioctadecyl-3,3,3 \ 3'-tetramethylindocarbocyanine perchlorate) and confocal laser scanning investigated the patterns of restoration of intermodular axon bonds in the zone of mechanical damage of the cerebral cortex against the background of embryonic nervous tissue and without it. It has been established that the introduction of embryonic neural tissue into the injury zone provides growth of axons that, after passing through the graft, connect to the adjacent brain tissue, whereas without transplantation of embryonic neural tissue, the damage zone is an insurmountable obstacle for growing axons. In this work transplantation of embryonic (15-17th day of gestation) of the neocortex was carried out. The results obtained by the authors are another evidence in favor of the active effect of the embryonic neural tissue graft on the posttraumatic reorganization of the inter-neuronal relationships of the neighboring structural and functional modules of the cerebral cortex. Transplantation of the embryonic nerve tissue provides partial restoration of the links between the damaged lesions of the cerebral cortex due to the creation of favorable conditions for the growth of axons in the zone of action of neurotrophic factors of the transplant. The existence of such an effect has been proved experimentally and is discussed in the literature as evidence of the high plastic capacities of the damaged brain of sexually mature animals. In this regard, cell transplantation is currently considered as an optimal therapeutic strategy for restoring the function of a damaged human CNS.
The data obtained by the authors on the effectiveness of the use of the embryonic neural tissue of the brain as an exogenous transplantation medium for the growth of axons serve as confirmation of the prospect of a purposeful creation of communication links between intact neighboring regions of the brain. The work on studying the effect of neural tissue transplantation on the dynamics of functional parameters of the central nervous system, whose task was to investigate the effect of locus coeruleus (LC) embryonic embryo transfer on the morphofunctional parameters of LC neurons and the locomotor activity of recipients, is relevant. Recipients were female Wistar rats, donors - 18-day-old embryos of rats of the same line. Transplantation of embryonic LC was carried out into the cavity of the third ventricle of the brain. Histologically, transplant engraftment was detected in 75% of the recipient animals. In cases of engraftment, the graft rested on the ventricle wall, filling 1 / 5-2 / 5 of its lumen, and was viable. At 1 and 6 months after the operation, the transplanted neural tissue according to morphological characteristics represented structures that would have arisen in their normal ontogenetic development, that is, the structure of the LC. The data obtained by the authors indicate that in animals that have been transplanted with the embryonic LC insert, the dynamic activity changes and the matrix activity of the chromatin of the LC cell nuclei increases. Consequently, intensification of the activity of the neurons of the own LC occurs, but the implant graft is also functionally active. It is known that the so-called locomotor region of the midbrain practically coincides with the localization of LC. The authors believe that the motility of rats receiving recipients is based on the activation of LC cells, both in-patient and transplant, with the release of a large amount of norepinephrine, including in the segments of the spinal cord. Thus, it is assumed that the increase in motor activity under conditions of LT transplantation into the intact brain of animals is due to the presence of a functionally active graft integrated with the recipient's brain and contributing to the activation of locomotor activity in rats.
In addition, transplanted neuroepithelial cells of the embryonic bookmark of the neocortex and spinal cord survive and differentiate into neuroblasts, young and mature neurons within 1-2 months after their transplantation into the injured sciatic nerve of sexually mature rats. In the study of the dynamics of the development of NADRN-positive neurons in embryonic neocortical and rat spinal cordmarks in heterotopic allografts (15-day-old rat embryos), engraftment of 70 to 80% of neurotransplants was revealed on longitudinal sections through sciatic nerve of the recipient rats, which depended on the observation time. Uniforms of unipolar and bipolar form with rounded light nuclei and one or two nucleoli began to form in transplants one week after the operation, which was accompanied by the formation of clusters. Among the neuroblasts, the authors failed to detect cells containing NADPH-diafopase (NADPH-d). After 7 days, only the cellular elements of the blood vessels - the endotheliocytes of the capillaries in the thickness of the graft, as well as the endothelial and smooth muscle cells of the vessels of the sciatic nerve of the recipient were NADPH-positive. Since the induction of NO-synthase (NOS) occurs in the smooth muscle cells of the vessels, IL-1, the authors associate the appearance of NADPH-positive smooth muscle cells in the blood vessels of the sciatic nerve with the presence of IL-1 synthesized in damaged nerve trunks. It is known that neuronogenesis in conditions of transplantation of embryonic brain deposits occurs synchronously with the development of neurons in situ. The results of morphological studies indicate that the differentiation of a part of the neural elements of transplants seven days after transplantation corresponds to the differentiation of cells of similar brain regions of newborn rats. Thus, under conditions of heterotopic transplantation into the peripheral nerve, transplanted nerve cells of the embryo show the ability to synthesize NADPH-d. At the same time, more neurons containing NADPH-d are found in the spinal cord transplants than in neocortex transplants, however, the synthesis of nitric oxide begins in transplanted neurons later than in in situ development. In the CNS of vertebrates, NOS-positive cells appear already in the prenatal period. It is believed that NO contributes to the formation of synaptic connections in the developing brain, and the presence of NOS-positive nerve afferent fibers that provide NO synthesis in the cerebellar neuroblasts stimulates the migration and differentiation of neurons, resulting in the formation of normal cytoarchitectonics of the brain. An important role of NO in synapsogenesis is established in the tectum - NOS-positive were only those neurons that had synaptic connections with the cells of the retina.
It is known that nitric oxide is one of the regulators of brain activity, where it is formed from arginine under the influence of NO synthase, which has diaphorous activity. In the CNS, N0 is synthesized in endothelial cells of blood vessels, microglia, astrocytes and in neurons of various parts of the brain. After traumatic brain damage, as well as hypoxia and ischemia, there is an increase in the number of neurons containing NO, which is one of the regulators of cerebral blood flow. Given the ability of N0 to induce synapsogenesis, the study of the formation of NO-containing cells in conditions of neurotransplantation on the background of traumatic injuries of the recipient's nervous tissue is of particular interest.
No less important is the study of the effect of neurotransplantation on the conditioned reflex stereotype of behavior. In experiments to study the effects of intrabrain and distant (between CII and CIII) tissue transplantation of the embryonic bluish spot (17-19 days gestation) on the processes of memory and catecholamine content in rats with destruction of the frontotemporal neocortex, it was shown that electrolytic damage to the frontotemporal the cerebral cortex disrupts the stereotype of the conditioned reflex emotional avoidance reaction (memory), weakens physiological activity, reduces the content of norepinephrine in the zone of the coagulated neocortex, but increases t its level in the hypothalamus, where there is a decrease in the concentration of adrenaline, although in the blood and adrenal glands its amount increases.
As a result of intracerebral grafting of embryonic bluish tissue in 81.4% of animals, the stereotype of the conditioned reflex emotional avoidance reaction, disturbed by electrolytic damage to the fronto-temporal parts of the cerebral cortex, normalizes the adrenaline content in the reticular formation of the midbrain, hypothalamus and neocortex, and in the hippocampus even its level rises, which is combined with a decrease in the concentration of adrenaline in the blood.
Distant transplantation of the tissue of the embryonic bluish spot contributes not only to restoration of the disturbed stereotype of the conditioned reflex emotional avoidance reaction in rats with electrolytic lesion of the frontotemporal cortex of the brain, but also increases the content of noradrenaline and adrenaline, mainly in the hypothalamus, blood, adrenals and heart. It is assumed that this is due to the vascularization of the graft, the penetration of neurotransmitters into the bloodstream, the passage through the blood-brain barrier and the activation of the mechanisms for the re-uptake of epinephrine and noradrenaline by uptake types 1, 2, 3. The authors believe that prolonged stabilization of the norepinephrine level under conditions of engraftment and functioning The transplant can be considered as a phenomenon of its progressive release in minimal doses by neurons of a bluish spot.
Positive clinical effects of embryonic neural tissue transplantation may be due to the ability of the latter to influence the processes of neoplasm of vessels, in the regulation of which growth factors and cytokines directly participate. Activates vasculogenesis of angiogenic growth factors - vascular endothelial growth factor (VEGF), FGF, PDGF and TGF, which are synthesized in ischemia, which is the initiating moment of angiogenesis. It is proved that the depletion potential of vascular growth occurs in the process of aging, which plays an important role in the pathogenesis of diseases such as coronary heart disease and obliterating atherosclerosis of the lower limbs. Ischemia of tissues develops and with a variety of other diseases. The introduction of angiogenic factors into ischemic zones (therapeutic angiogenesis) stimulates the growth of blood vessels in ischemic tissues and improves microcirculation due to the development of collateral circulation, which in turn increases the functional activity of the affected organ.
The most promising for clinical use are VEGF and FGF. The results of the first randomized trials proved to be encouraging, especially provided the correct choice of the optimal dosages and modes of administration of angiogenic factors. In this connection, an experimental evaluation of the angiogenic activity of an extract isolated from human embryonic brain tissue has been carried out. The work used abortion material obtained at the twentieth week of pregnancy and processed according to the method of I. Maciog and co-authors (1979) in the modification of the ANRF IC. This drug is an analogue of "Endothelial cell growth supplement" ("Sigma") and is a natural mixture of human angiogenic factors, which includes VEGF and FGF. The experiments were performed on rats with models of ischemia of the tissue of the hind limb and the myocardium. Based on the study of alkaline phosphatase activity in experimental animals receiving an extract of embryonic nerve tissue, an increase in the number of capillaries per unit area of the myocardium, both in longitudinal and transverse sections of the heart, was revealed. The angiogenic activity of the drug was manifested with direct introduction into the ischemia zone, as well as in the case of systemic (intramuscular) administration, which led to a decrease in the average area of postinfarction cicatrix.
In any variant of embryonic neural tissue transplantation, it is extremely important to correctly select the gestational age of the transplanted embryonic material. A comparative analysis of the efficacy of cell preparations from embryonic ventral mesencephalon of 8-, 14- and 16-17-day-old embryos of rats three months after intra-pace neurotransplantation to mature rats with parkinsonism in an automated test of apomorphine-induced motor asymmetry revealed a significantly higher efficiency of CNS cellular preparations of 8-day embryos and the smallest - from a 16-17 day old embryonic nerve tissue. The obtained data correlated with the results of histomorphological analysis, in particular, with the sizes of grafts, the degree of glial reaction and the number of dopaminergic neurons in them.
Differences in the therapeutic effect of embryonic neural tissue cells can be related both to the degree of immaturity and committalization of the cells themselves, and to their different responses to growth factors that are released in the zone of induced damage to dopaminergic neurons. In particular, the effect of EGF and FGF2 on the development of neural stem cells of telencephalon in vivo occurs at different stages of embryogenesis. Neuroepithelial cells of 8.5-day-old mouse embryos when cultured in vitro in a serum-free medium proliferate in the presence of FGF2, but not EGF, to which only stem cell populations isolated from the embryo brain at a later stage of development react. At the same time, neural stem cells proliferate in response to each of these mitogens and additively increase growth when EGF and FGF2 are added to a culture with a low cell density. It is believed that the EGF-reactive neural stem cells of the embryonic zones of 14.5-day-old mouse embryos are linear descendants of FGF-reactive neural stem cells that first appear after 8.5 days of gestation. The potential phenotype of neural stem and progenitor cells depends on the complex effect of their microenvironment. Immunophenotyping of neural cells of the periventricular and hippocampal zones of 8-12- and 17-20-week human embryos by flow cytofluorometry revealed significant variability, related both to the gestation period and to individual constitutional features of the donor biomaterial. When these neural progenitor cells are cultured in a selective serum-free medium with EGF, FGF2, and NGF, neurospheres are formed at a rate substantially dependent on the gestation period. Cells from different parts of the brain of a 5-13 week old human embryo during short-term cultivation with FGF2 in monolayer culture on a laminin substrate in the presence of trace amounts of growth factors support proliferation for 6 weeks with a high percentage of non-stinging cells against spontaneous cell formation with markers of all three lines neural differentiation. Cells isolated from the human mesencephalon at a gestation period exceeding 13 weeks proliferate under the influence of EGF and also form neurospheres. Thanks to the combination of EGF and FGF2, a synergistic effect was achieved. The most intensive proliferation of neural stem cells with the emergence of neurospheres is observed when culturing the cerebral cortex tissue of 6-8-week-old human embryos in the presence of EGF2, IGF1 and 5% horse serum on a fibronectin substrate.
It should be noted that questions concerning the gestational age and the department of the embryonic CNS, the tissue of which is preferable to use for the purpose of neurotransplantation, remain open. Answers to them should be sought in the neurogenesis of the developing brain, which continues throughout the prenatal period - at a time when the epithelium of the neural tube forms a multilayered structure. It is believed that the source of stem cells and new neurons is the radial glia consisting of elongated cells with long processes radially directed to the wall of the cerebral blisters and in contact with the inner surface of the ventricles and the external pial surface of the brain wall. Earlier, radial glia was only endowed with a function of the neuronal tract, through which the neuroblasts migrated from the ventral region to the surface areas, and also assigned to it a skeletal role in the formation of the correct laminar organization of the cortex. Today it is established that as the development of radial glia is transdifferentiated into astrocytes. A significant part of it in mammals is reduced immediately after birth, however, in those species of animals in which radial glia persists to adulthood, neuronogenesis also actively proceeds in the postnatal period.
In the culture of radial glial cells, neurons and glial cells were formed from the embryonic neocortex of rodents, and neurons predominantly formed on the gestational age of embryos from 14 to 16 days (the period of maximum intensity of neuronogenesis in the cerebral cortex of mice and rats). On the 18th day of embryogenesis, differentiation shifted toward astrocyte formation with a significant decrease in the number of newly formed neurons. In situ marking of radial glial cells with GFP made it possible to detect asymmetric division of labeled cells in the brain bubble cavity of 15-16 day old rat embryos with the appearance of daughter cells with immunological and electrophysiological characteristics of neuroblasts. It is remarkable that, according to the results of dynamic observations, the emerging neuroblasts use the mother cell of radial glia for migration to the pial surface.
The endogenous marker of radial glia is the protein of intermediate neustin filaments. Fluorescent flow sorting of cells labeled with a retrovirus associated with GFP and expressed under the control of nestin showed that the stem cells of the dentate gyrus and hippocampus chimpus region (material was obtained in epilepsy operations) express nestin. Consequently, they refer to the radial glia, which in humans, as in other mammals, is retained only in the dentate gyrus.
At the same time, the effectiveness of cell transplantation is determined not only by the high viability of donor cells, their differentiation potential and the property of replacing defective cells, but, first of all, by directed migration. It is from the migration ability that the full functional integration of the transplanted cells depends - without disturbances in the cytoarchitectonics of the recipient brain. Since the radial glia in the postnatal period is almost completely reduced, it was necessary to find out how in the adult recipients the donor cells can move from the transplantation zone to the lesion focus of the brain. There are two variants of cell migration in the central nervous system, independent of radial glia: the phenomenon of tangential migration or the movement of neuroblasts in the development of the cerebral cortex perpendicular to the radial glial network, and migration by a string or chain. In particular, the migration of neural progenitor cells from the rostral subventricular zone to the olfactory bulb occurs as a sequence of tightly adhering cells surrounded by glial cells. It is believed that these cells use partner cells as a migration substrate, and the main regulator of such intercellular interactions is PSA-NCAM (polysialized molecule of adhesion of nerve cells). Consequently, migration of neurons does not necessarily require the participation of radial glia or pre-existing axonal bonds. The pernatal form of cell migration by the "string" along the rostral migratory pathway is maintained throughout life, which indicates a real possibility of targeted delivery of transplanted neural progenitor cells into the mature nervous system.
There is a hypothesis about the presence of a stem cell line in the ontogenesis of the brain, according to which in the early stages of brain development the stem cell is the neuroepithelial cell, which, as it matures, is transdifferentiated into radial glia. In adulthood, the role of stem cells is performed by cells that have signs of astrocytes. Despite a number of controversial issues (the controversy over stem cells of the hippocampus, as well as the deep divisions of the brain that do not have a layered structure of the cortex and developing from thalamic tubercles, where radial glia is absent), a clear and simple concept of a consistent change in the phenotype of stem cells throughout ontogeny looks very attractive.
The effect of microenvironment factors on the determination and subsequent differentiation of neural differential cell cells is clearly demonstrated in the transplantation of stem cells of the mature rat spinal cord into different parts of the mature nervous system. When stem cells were transplanted into the dentate gyrus or into the area of migration of neurons of olfactory bulbs, active transplantation of the cells to numerous neurons was observed. The transplantation of stem cells into the spinal cord and the region of the ammon horn led to the formation of astrocytes and oligodendrocytes, whereas during transplantation not only glial cells but also neurons were formed in the dentate gyrus.
In a sexually mature rat, the number of dividing cells in the dentate gyrus can reach several thousand per day - less than 1% of the total number of grain cells. Neurons account for 50-90% of cells, astrocytes and other glial elements - about 15%. The remaining cells do not have antigenic signs of neurons and glia, but they contain antigens of endothelial cells, which indicates a close relationship between neuronogenesis and angiogenesis in the dentate gyrus. Proponents of the possibility of differentiating endothelial cells into neuronal progenitor cells refer to the ability of endotheliocytes in vitro to synthesize BDNF.
Impressive speed of self-assembly of neural circuits: in the process of differentiation, the progenitor cells of the grain cells migrate into the dentate gyrus and form processes growing toward the zone of the CAS ammon horn and forming synapses with pyramidal glutamatergic and intercalating inhibitory neurons. The newly created grain cells are built into existing neural circuits within 2 weeks, and the first synapses appear already on the 4th-6th day after the appearance of new cells. By the frequent administration of BrdU or 3H-thymidine to a mature animal (one of the methods for identifying adult stem cells), a large number of labeled neurons and astrocytes in the ammonoid horn have been detected, which indicates the possibility of the formation of new neurons, not only in the dentate gyrus, but also in other parts of the hippocampus. Interest in the processes of division, differentiation and death of cells in the dentate gyrus of the hippocampus of the mature brain is also due to the fact that the neurons that form here are localized in one of the key places of the hippocampus, responsible for learning and memory processes.
So, it is now established that from the cells of the subendendymic zone of the lateral ventricle of mature rodents, neural precursor cells migrate along the rostral migratory path formed by longitudinally oriented astrogal cells to the olfactory bulb, where they are inserted into the layer of grain cells and differentiate into neurons of this structure. Migration of progenitorial neural cells is revealed in the rostral migratory tract of adult monkeys, which indicates the possibility of the formation of new neurons in the olfactory bulb of primates. Neural stem cells are isolated from the olfactory bulb of an adult and transferred to lines whose cloned cells differentiate into neurons, astrocytes and oligodendrocytes. Stem cells are found in the hippocampus of the mature brain of rats, mice, monkeys and humans. Neural stem cells of the subgrainular zone of the dentate fascia are the source of progenitor cells migrating into the medial and lateral limbs of the hippocampus, where they differentiate into mature cells-grains and glial elements. The axons of de novo-formed neurons of the dentate fascia are traced to the CAS field, which indicates the participation of newly formed neurons in the realization of the functions of the hippocampus. In the associative regions of the new cerebral cortex of adult monkeys, progenitor cells of neurons migrating from the subventricular zone were found. In the sixth layer of the new cerebral cortex of mice, new pyramidal neurons are revealed 2-28 weeks after the induced damage and death of the native neurons of this layer due to the migration of previously dermant precursor cells of the subventricular zone. Finally, the reality of postnatal neurogenesis in the human brain is evidenced by a twofold increase in the number of cortical neurons, which lasts for the first 6 years after birth.
Of no small importance for practical cell transplantation is the question of the regulation of the processes of reproduction and differentiation of neural stem and progenitor cells. The most important among the factors inhibiting proliferation of neural progenitor cells are glucocorticoids, which sharply reduce the number of divisions, whereas adrenal removal, by contrast, significantly increases the number of mitoses (Gould, 1996). It is noteworthy that the morphogenesis of the dentate gyrus in rodents is most intense during the first two weeks of postnatal development in the period when there was no reaction to stress on the background of a sharp decrease in production and secretion of steroid hormones of the adrenal cortex. Corticosteroids inhibit the migration of cell-grains - new neurons do not integrate into the granular layer of the dentate gyrus, but remain in the chylus. It is assumed that the processes of synaptic bond formation are simultaneously violated. Protection of cells from such "steroid aggression" is carried out by minimally expressing mineral- and glucocorticoid receptors on proliferating cells-grains, not only during the development of the dentate gyrus, but also in mature animals. Nevertheless, of all neurons in the brain, it is the hippocampal neurons that are characterized by the highest content of glucocorticoid receptors, which causes a stressful effect on the hippocampus. Psychoemotional stress and stressful situations inhibit neuronogenesis, and chronic stress dramatically reduces the ability of animals to learn new skills and learn. The more pronounced negative effect of chronic stress on neuronogenesis is quite understandable, given the predominantly dormant state of neural stem cells. When immobilizing pregnant rats (for the rodent - an extremely strong stress factor), it is established that prenatal stress also causes a decrease in the number of cells in the dentate gyrus and substantially inhibits neuronogenesis. It is known that glucocorticoids take part in the pathogenesis of depressive states, the morphological equivalent of which is the inhibition of neuronogenesis, pathological reconstruction of neurons and neuronal connections, and the death of nerve cells. On the other hand, antidepressant chemotherapy drugs activate de novo neuronal formation, which confirms the connection between the processes of formation of new neurons in the hippocampus and the development of depression. Essential effect on neuronogenesis is exerted by estrogens, the effects of which are opposite to the action of glucocorticosteroids and are to support the proliferation and viability of neural progenitor cells. It should be noted that estrogens significantly increase the ability of animals to learn. Some authors with the influence of estrogens associate cyclic changes in the number of cells-grains and exceeding their number in females.
It is known that neurogenesis is controlled by EGF, FGF and BDNF, but the mechanisms of the effect of external signals on stem cells from mitogens and growth factors have not been studied enough. It was found that PDGF in vitro supports the neuronal orientation of progenitor cell differentiation, and the ciliary neurotrophic factor (CNTF), like triiodothyronine, stimulates the formation of predominantly glial elements - astrocytes and oligodendrocytes. Pituitary adenylate cyclase-activating protein (PACAP) and vasoactive intestinal peptide (VIP) activate proliferation of neural progenitor cells, but inhibit the processes of differentiation of daughter cells. Opioids, especially in the case of prolonged exposure, significantly inhibit neuronogenesis. However, the stem cells and neural progenitor precursor cells of the dentate gyrus do not have opioid receptors (they are present in differentiating neurons of the embryonic period), which does not allow to evaluate the direct effects of opioids.
The needs of practical regenerative and plastic medicine forced researchers to pay special attention to the study of pluri- and multipotency of stem cells. Realization of these properties at the level of the regional stem cell of an adult organism in the long term could ensure the development of the necessary transplantation material. It has been shown above that epigenetic stimulation of neural stem cells allows obtaining proliferating cells already preformed according to neural phenotypes, which limits their number. In the case of using the totipotent properties of the embryonic stem cell, proliferation until a sufficient number of cells is obtained occurs before neural differentiation, and the expanded cells are easily converted into a neural phenotype. To obtain neural stem cells, ESK is extracted from the internal cell mass by blastocysts and cultured with the obligatory presence of LIF, which preserves their totipotency and the ability to unrestricted division. After that, retinoic acid is induced by neural differentiation of ESC. Transplantation of the neural stem cells thus obtained into a striatum damaged by quinoline and 6-hydroxydopamine is accompanied by their differentiation into dopaminergic and serotonergic neurons. After introduction into the ventricles of the rat embryo brain, the neural progenitor cells derived from the ESC are migrated to various regions of the recipient's brain, including the cortex, striatum, septum, thalamus, hypothalamus and cerebellum. Cells remaining in the cavity of the ventricles form epithelial structures resembling a neural tube, as well as individual islets of non-neural tissue. In the parenchyma of the brain of the recipient embryo, the transplanted cells produce three main types of cells in the nervous system. Some of them have elongated apical dendrites, pyramidal cell bodies and basal axons projecting into a corpus callosum. Astrocytes of donor origin extend the processes to the nearby capillaries, and the oligodendrocytes are in close contact with the myelin clutches, taking part in the formation of myelin. Thus, neural progenitor cells derived from ESC in vitro are capable of directional migration and adequate microenvironmental signals to regional differentiation, providing many areas of the developing brain with neurons and glia.
Some authors consider the possibility of de-and transdifferentiation of regional stem cells of an adult organism. An indirect confirmation of the dedifferentiation of cells in culture with the expansion of their potencies is data on the engraftment of stem neural cells of mice in the red bone marrow, followed by the development of cell lines from them, which give functionally active cells of peripheral blood. In addition, the transplantation of genetically labeled (LacZ) cells from neurospheres derived from the mature or embryonic brain into the brain of irradiated mice with oppressed hematopoies led to the formation of not only neural derivatives from stem cells, but also caused the generation of blood cells, which indicates the pluripotency of the neural stem cells, realized outside the brain. Thus, the neural stem cell is able to differentiate into blood cells under the influence of the signals of the microenvironment of the bone marrow with a preliminary transformation into the hematopoietic stem cell. On the other hand, during the transplantation of bone marrow stem cells into the brain, their differentiation was established under the influence of the microenvironment of brain tissue in glial and nerve cells. Consequently, the differentiating potential of nerve and hematopoietic stem cells is not limited by tissue specificity. In other words, the factors of the local microenvironment, other than those characteristic of the tissues of the brain and bone marrow, can change the direction of differentiation of these cells. It is shown that neural stem cells introduced into the venous system of irradiated mice create a population of myeloid, lymphoid and immature hematopoietic cells in the spleen and bone marrow. In vitro, the effect of bone marrow morphogenetic proteins (BMPs) on the survival and differentiation of neural stem cells has been established, determining, as in the early stages of embryogenesis, their development in the neural or glial directions. In cultures of neural stem cells, 16-day-old embryo rats, BMPs induce the formation of neurons and astroglia, whereas in cultures of stem cells derived from the perinatal brain, only astrocytes are formed. In addition, BMPs suppress the generation of oligodendrocytes, which in vitro appear only when the antagonist BMPs of the noggin is added.
The processes of transdifferentiation are inherent in vidonesspecificity: human hematopoietic stem cells in the bone marrow transplanted into the striatum of sexually mature rats migrate to the white matter of the outer capsule, the ipsi and contralateral neocortex, where they form astrocyte-like cellular elements (Azizi et al., 1998). In the allotransplantation of bone marrow stem cells into the lateral ventricle of newborn mice, the migration of hematopoietic stem cells can be traced to the structures of the forebrain and the cerebellum. In the striatum and the molecular layer of the hippocampus, the migrated cells are transformed into astrocytes, and in the olfactory bulb, the inner layer of cerebellar cells and the reticular formation of the brainstem, neuron-like cells form with a positive response to neurofilaments. After intravenous administration to adult mice of hematopoietic cells, GFP-labeled micro- and astrocytes are detected in the neocortex, thalamus, brainstem and cerebellum.
In addition, mesenchymal stem cells of the bone marrow, which give rise to all types of cells of connective tissue, under certain conditions may also undergo neural transdifferentiation (recall that the neural crest cells are the embryonic source of the mesenchyme). It has been shown that human and mouse bone marrow stromal cells cultured in vitro in the presence of EGF or BDNF express the marker of neural neustin precursor cells, and the addition of various combinations of growth factors leads to the formation of cells with glial markers (GFAP) and neurons (nuclear protein, NeuN). Labeled syngenic mesenchymal stem cells transplanted into the lateral ventricle of the brain of newborn mice migrate and localize in the forebrain and the cerebellum without violating the cyto-architectonics of the recipient brain. Mesenchymal bone marrow stem cells differentiate into mature astrocytes in the striatum and molecular layer of the hippocampus, and also colonize the olfactory bulb, the granular layers of the cerebellum and the reticular formation, where they are converted into neurons. Mesenchymal stem cells from human bone marrow can in vitro differentiate into macroglia, and after transplantation integrate into the structures of the rat brain. Direct transplantation of mesenchymal bone marrow stem cells into the hippocampus of adult rats is also accompanied by their migration to the parenchyma of the brain and neuroglial differentiation.
It is assumed that the transplantation of bone marrow stem cells can expand the possibilities of cell therapy for CNS diseases characterized by excessive pathological death of neurons. It must be noted, however, that not all researchers recognize the fact of mutual transformation of neural and hematopoietic stem cells, especially in in vivo conditions, which is again due to the lack of a reliable marker for evaluating their transdifferentiation and further development.
Stem cell transplantation opens up new horizons for the cellular gene therapy of hereditary neurological pathology. The genetic modification of neural stem cells involves the integration of genetic regulatory constructs, whose products interact with the proteins of the cell cycle in the regime of automatic regulation. The transduction of such genes into embryonic progenitor cells is used to multiply neural stem cells. Most genetically modified cell clones behave like stable cell lines without showing signs of transformation in vivo or in vitro, but has a pronounced ability to contact inhibition of proliferation. When transplanting multiplied transfected cells, the latter are inserted into the tissue of the recipient, without violating cytoarchitectonics and without undergoing a tumor transformation. Donor neural stem cells do not deform the integration zone and compete equitably for space with the progenitor cells of the host. However, on the 2nd-2nd day, the intensity of division of transfectant cells decreases sharply, which corresponds to contact inhibition of their proliferation in vitro. In embryos-recipients of neural stem transfectants, there are no abnormalities of the development of the central nervous system, all areas of the brain that contact the graft develop normally. After transplantation, clones of neural stem cells migrate rapidly from the zone of administration and often go beyond the corresponding germinal zones along the rostral tract, adequately integrating with other areas of the brain. The incorporation of genetically modified clones and transfected cell lines of neural stem cells into the brain of the host organism is characteristic not only for the embryonic period: these cells are implanted in numerous areas of the central nervous system of the fetus, newborn, adult and even aging organism of the recipient and, at the same time, differentiation. In particular, after transplantation into the cerebral cavity of the brain, transfected cells migrate without damaging the blood-brain barrier and become integral functional cellular components of brain tissue. Donor neurons form the appropriate synapses and express specific ion channels. While maintaining the integrity of the blood-brain barrier, astroglia, a derivative of the neural stem transfection cells, extends the processes to the cerebral vessels, and oligodendrocytes of donor origin express the main myelin protein and myelinate the processes of the neurons.
In addition, neural stem cells are transfected for use as cellular vectors. Such vector-genetic constructs provide in vivo stable expression of foreign genes involved in the development of the nervous system or are used to correct existing genetic defects, since the products of these genes are able to replenish various biochemical abnormalities of the central nervous system. High migration activity of transfected stem cells and adequate implantation in the embryonic zones of various regions of the developing brain allow us to hope for a complete restoration of the hereditary deficit of cellular enzymes. In the modeling of ataxia-telangiectasia syndrome (mutant lines of pg and pcd mice) Purkinje cells disappear from the cerebellum of experimental animals during the first weeks of postnatal development. It is shown that the introduction of neural stem cells into the brain of such animals is accompanied by their differentiation into Purkinje cells and granular neurons. In pcd mutants, the coordination of movements is partially corrected and the intensity of tremor decreases. Similar results were obtained in the transplantation of cloned human neural stem cells to primates in which Purkinje cell degeneration was induced by oncanase. After transplantation, donor neural stem cells were found in the granular and molecular layers, as well as in the Purkinje cell layer of the cerebellar parenchyma. Therefore, the genetic modification of neural progenitor cells is capable of providing a stable, committed modification of the phenotype that is resistant to external influences. This is especially important in pathological processes associated with the development in the recipient of factors hindering the survival and differentiation of donor cells (for example, with immune aggression).
Mucopolysaccharidosis type VII in humans is characterized by neurodegeneration and progressive retardation of intellectual development, which in the mouse experiment is modeled by a deletion mutation of the beta-glucuronidase gene. After transplantation into the ventricles of the brain of newborn defective recipient mice transfected neural stem cells secreting beta-glucuronidase, the donor cells are detected first in the terminal zone, and then spread through the brain parenchyma, stably correcting the integrity of the lysosomes in the brain of the mutant mice. In the Tay-Sachs disease model, retrovirus-transfected neural stem cells with intrauterine introduction into the fetus of mice and transplantation to newborn mice provide for effective expression of beta-subunit beta-hexosaminidase in recipients with a mutation leading to abnormal accumulation of beta2-ganglioside.
Another direction of regenerative medicine is the stimulation of the proliferative and differentiating potential of the own neural stem cells of the diseased organism. In particular, neural stem cells secrete NT-3 in the hemisection of the spinal cord and brain asphyxia in rats, express NGF and BDNF in septum and basal ganglia, tyrosine hydroxylase in the striatum, as well as rilin in the cerebellum and the main myelin protein in the brain .
However, there is clearly insufficient attention to the stimulation of neuronogenesis. A few works give grounds to believe that the functional load on the nerve centers responsible for distinguishing odors is reflected in the formation of new neurons. In transgenic mice with a deficiency of neuronal adhesion molecules, a decrease in the intensity of neuronogenesis and a decrease in the number of neurons migrating to the olfactory bulbs was combined with a disruption in the ability to distinguish smells, although the odor threshold and short-term olfactory memory were not violated. In the regulation of neuronogenesis, the functional state of the dentate gyrus cells plays an important role: weakening the effect of glutamate on the grain cells after the destruction of the entorhinal cortex promotes the proliferation and differentiation of neurons, and the stimulation of the fibers of the perforating pathway (the main afferent entry into the hippocampus) causes inhibition of neuronogenesis. NMDA receptor antagonists activate neuronal neoplasm processes, whereas agonists, on the contrary, reduce the intensity of neuronogenesis, which in effect resembles the action of glucocorticosteroids. In the literature there are conflicting research results: the information on the experimentally proven inhibitory effect of the excitatory neurotransmitter glutamate on neuronogenesis does not agree with the data on the stimulation of the progenitor cells and the appearance of new neurons with increased convulsive activity in the hippocampus of animals with experimental kain and pilocarpine models of epilepsy. At the same time, on the traditional model of epilepsy caused by multiple subthreshold stimulation of a specific region of the brain (Kindling) and characterized by less pronounced neuronal death, the intensity of neuronogenesis increases only in the late phase of the kindling, when damage to and death of neurons is observed in the hippocampus. It is shown that with epilepsy convulsive activity stimulates neuronogenesis with abnormal localization of new granular neurons, many of which appear not only in the dentate gyrus, but also in the chylus. Such neurons are of great importance in the development of the scaffolding of moss-like fibers, since their axons form inverse collateralals, which are not normally normal, forming numerous synapses with neighboring grain cells.
The use of regional neural stem cells opens new prospects for the use of cellular transplantation in the therapy of metabolic and genetic neurodegenerative diseases, demyelinating diseases and post-traumatic disorders of the CNS functions. Before carrying out the substitution cell transplantation, one of the methods selects and expands the necessary type of neural progenitor cells ex vivo with the purpose of their subsequent introduction directly into the damaged area of the brain. The therapeutic effect in this case is due to replacement of damaged cells or local release of growth factors and cytokines. This method of regenerative-plastic therapy requires the transplantation of a sufficiently large number of cells with predefined functional characteristics.
Further studies on the molecular characteristics and regenerative-plastic potencies of the mature brain stem cells, as well as the ability to transdifferentiate regional stem cells of different tissue origin, should also be considered appropriate. Today, a screening of antigens of the hematopoietic stem cells of the bone marrow has been carried out to determine the marker combination of cells capable of being transdifferentiated into neural stem progenitor cells (CD 133+, 5E12 +, CD34-, CD45-, CD24). Cells that form in vitro neurospheres and form neurons are obtained during transplantation into the brain of newborn immunodeficient mice. Interest in cell xenotransplantology is the result of studies on the possibility of cross-stem cell transplantation in individuals of evolutionarily distant taxa. So far, the results of the implantation of neural stem cells into the brain tumor region remain without proper interpretation: the transplanted cells actively migrate throughout the volume of the tumor without going beyond it, and when cells are introduced into the intact part of the brain, they are actively migrating towards the tumor. The question of the biological significance of such migration remains open.
It should be noted that successful transplantation of neural stem cells, as well as of other neural progenitor cells obtained from ESC, is possible only under conditions of using highly purified neural progenitor cells, since undifferentiated embryonic stem cells during transplantation to an adult immunocompetent recipient inevitably transform into teratomas and teratocarcinomas. Even the minimal amount of low-grade cells in the donor cell suspension dramatically increases the tumorigenic nature of the graft and unacceptably increases the risk of tumors or the formation of non-neural tissue. The production of homogeneous populations of neural progenitor cells is possible by using cells that originate at certain stages of normal embryogenesis as an alternative source of donor tissue. Another approach is to carefully eliminate unwanted cell populations by line-specific selection. The danger also represents application for the purpose of neurotransplantation of ESC after their insufficient exposure in vitro with growth factors. In this case, the program of neural differentiation can not be excluded with the formation of structures inherent in the neural tube.
Today it is quite obvious that neural stem cells exhibit tropism to pathologically altered areas of the central nervous system and have a pronounced regenerative-plastic effect. The microenvironment in the lesion of neural tissue cells models the direction of differentiation of transplanted cells, thus replenishing the deficit of specific neural elements within the CNS lesion zone. In some neurodegenerative processes, neurogenic signals appear to recapitulate the neuronogenesis, and the neural stem cells of the mature brain are able to respond to this instructive information. A clear illustration of the therapeutic potential of neural stem cells is the numerous data from experimental studies. Intracisternal administration of clone of neural stem cells to animals with ligation of the middle cerebral artery (ischemic stroke model) contributes to a decrease in the area and volume of the destructively altered region of the brain, especially in the case of neural stem cell transplantation together with FGF2. Immunocytochemically, migration of donor cells into the ischemic zone is observed, followed by their integration with intact brain cells of the recipient. Transplantation of immature cells of the neuroepithelial line of the MHP36 mouse in the rat brain during an experimental stroke improves the sensorimotor function, and the introduction of these cells into the ventricles of the brain enhances the cognitive function. As a result of transplantation to rats of neural-preformed hemopoietic cells of the human bone marrow, violations of the function of the cerebral cortex caused by ischemic damage are eliminated. In this case, the xenogeneic neural progenitor cells migrate from the site of injection into the zone of destructive changes in brain tissue. Intracranial transplantation of homologous bone marrow cells in traumatic brain cortex damage in rats results in partial restoration of motor function. The donor cells are implanted, proliferated, undergo neural differentiation into neurons and astrocytes, and migrate towards the lesion focus. When introduced into the striatum of adult rats with an experimental stroke, cloned human neural stem cells replace the damaged cells of the central nervous system and partially restore impaired brain function.
Human neuronal stem cells are predominantly isolated from embryonic telencephalon, which develops significantly later than the more caudal nerve trunk regions. The possibility of isolating neural stem cells from the spinal cord of the 43-137-day-old fetus is shown, since in the presence of EGF and FGF2 these cells form neurospheres and in the early passages exhibit multipotency, differentiating into neurons and astrocytes. However, prolonged cultivation of neural progenitor cells (over 1 year) deprives them of multipotency - these cells are able to differentiate only into astrocytes, that is, they become unipotent. Regional neural stem cells can be obtained as a result of partial bulbectomy and after multiplication in culture in the presence of LIF it is transplanted to the same patient with neurodegenerative changes in other parts of the central nervous system. In the clinic, replacement cell therapy with the use of neural stem cells was first performed for the treatment of patients with stroke accompanied by damage to the basal ganglia of the brain. As a result of transplantation of donor cells, the clinical state of most patients has improved.
Some authors believe that the ability of neural stem cells to survive, migrate and integrate into different areas of the nervous tissue with damage to the central nervous system opens up unlimited possibilities for cell therapy not only local, but also extensive (stroke or asphyxia), multifocal (multiple sclerosis) and even global ( most inherited metabolic disorders or neurodegenerative dementia) pathological processes. Indeed, in the transplantation of cloned mouse and human neural stem cells, to recipient animals (mice and primates, respectively) with degeneration of dopaminergic neurons in the mesostrial system induced by methyl-phenyl tetrapyridine (Parkinson's model of the disease) 8 months before transplantation, donor neural stem cells are integrated into the recipient's CNS. A month later the transplanted cells localize bilaterally along the midbrain. Some of the generated neurons of donor origin express tyrosine hydrolase in the absence of signs of an immune response to the graft. In rats injected with 6-hydroxydopamine (another experimental model of Parkinson's disease), the adaptation of transplanted cells to the microenvironment in the brain of the host was determined by the conditions of culturing the neural stem cells prior to their transplantation. Neural stem cells that proliferate rapidly in vitro under the influence of EGF, replenished the deficit of dopaminergic neurons in the injured striatum more effectively than cells from 28-day cultures. The authors believe that this is due to the loss of the ability to perceive the corresponding differentiation signals during the cell division of neural progenitor cells in vitro.
In separate works, attempts were made to increase the effectiveness of the effect on the reinnervation of a damaged striatum by transplanting the cells of the embryonic striatum into this region as a source of neurotrophic factors with simultaneous transplantation of dopaminergic neurons of the ventral mesencephalon. As it turned out, the effectiveness of neurotransplantation largely depends on the method of embryonic nerve tissue insertion. As a result of research on the transplantation of preparations of embryonic nerve tissue into the ventricular system of the brain (to avoid trauma of the striatum parenchyma), information has been obtained on their positive effect on the motor defect in Parkinsonism.
However, in other studies, experimental observations have shown that transplantation in the ventricle of the brain of ventral mezencephalon embryonic nerve tissue containing dopaminergic neurons, as well as transplantation of GABA-ergic embryonic neural elements into the striatum of rats with hemiparkinsonism, does not contribute to the restoration of impaired functions of the dopaminergic system. In contrast, immunocytochemical analysis confirmed the low survivability of dopaminergic neurons of ventral mesencephalon transplanted into the striatum of rats. The therapeutic effect of intraventricular transplantation of the embryonic nerve tissue of the ventral mesencephalon was realized only under the condition of simultaneous implantation of the preparation of embryonic striatal cells into the denatured striatum. The authors believe that the mechanism of this effect is associated with the positive trophic influence of GABA-ergic elements of the embryonic striatum on the specific dopaminergic activity of intraventricular ventral mesencephalon transplants. The expressed glial reaction in the grafts was accompanied by a slight regression of the apomorphine test. The latter, in turn, correlated with the content of GFAP in the blood serum, which directly indicated a violation of the permeability of the blood-brain barrier. Based on these data, the authors concluded that the serum GFAP level can be used as an adequate criterion for assessing the functional state of the graft, and the increased permeability of the blood-brain barrier for neurospecific antigens such as GFAP is a pathogenetic link in the development of graft failure due to autoimmune damage to the recipient's nerve tissue .
From the point of view of other researchers, engraftment and integration of neural stem cells after transplantation are stable and lifelong, since donor cells are found in recipients for at least two years after transplantation and without a significant reduction in their number. Attempts to explain this by the fact that, in an undifferentiated state, neural stem cells do not express MHC molecules of classes I and II at a level sufficient to induce an immune rejection reaction, it can be considered valid only with respect to low-grade neural precursors. However, not all neural stem cells in the brain of the recipient persist in an immatureodermant state. Most of them undergo differentiation, during which MHC molecules are expressed in full.
In particular, the insufficient effectiveness of the use of intravenous transplantation of embryonic ventral mesencephalon preparations containing dopaminergic neurons to treat experimental parkinsonism is associated with a low survival rate of transplanted dopaminergic neurons (only 5-20%), which is caused by reactive gliosis accompanying local trauma of the parenchyma of the brain at transplantation. It is known that local trauma of the parenchyma of the brain and concomitant gliosis lead to a violation of the integrity of the blood-brain barrier with the release of peripheral blood antigens of nervous tissue, in particular OKAR and neuron-specific antigen. The presence of these antigens in the blood can cause the production of specific cytotoxic antibodies to them and the development of autoimmune aggression.
V. Tsymbalyuk and co-authors (2001) report that the traditional view that the CNS is an immunologically privileged zone isolated from the immune system by the blood-brain barrier remains in force. In their review of the literature, the authors refer to a number of studies that suggest that this view does not fully correspond to the essence of immune processes in the brain of mammals. It is established that the labeled substances introduced into the parenchyma of the brain can reach deep cervical lymph nodes, and after intracerebral injection of antigens, specific antibodies are formed in the body. Cells of cervical lymph nodes correspond to proliferation to such antigens, starting from the 5th day after the injection. The formation of specific antibodies was also revealed in the transplantation of the skin into the parenchyma of the brain. The authors of the review give several possible ways of transporting the antigen from the brain to the lymphatic system. One of them is the transition of antigens from the perivascular spaces to the subarachnoid space. It is assumed that perivascular spaces, localized along large cerebral vessels, are equivalent to the lymphatic system in the brain. The second way lies along the white fibers - through the latticed bone into the lymphatic vessels of the nasal mucosa. In addition, there is an extensive network of lymphatic vessels in the dura mater. The blood-cell barrier for lymphocytes is also very relative. It is proved that activated lymphocytes are able to produce enzymes that affect the permeability of the structures of the "immune filter" of the brain. At the level of post-capillary venules activated T-helpers penetrate and through the intact blood-brain barrier. The thesis about the absence of cells representing the antigen in the brain does not stand up to criticism. At present, the possibility of representing antigens in the central nervous system by at least three types of cells has been convincingly proven. First, they are dendritic cells of bone marrow origin, which are localized in the brain along large blood vessels and in white matter. Secondly, antigens are able to present endothelial cells of the blood vessels of the brain, and in association with MHC antigens, which supports the clonal growth of T-cell specific antigens. Third, micro- and astroglia cells act as antigen-presenting agents. Participating in the formation of an immune response in the central nervous system, astrocytes acquire the properties of an immune-effector cell and express a number of antigens, cytokines and immunomodulators. When incubated with y-INF, astroglial cells in vitro express MHC class I and II antigens, and stimulated astrocytes are capable of antigenic representation and maintenance of clonal proliferation of lymphocytes.
Traumatization of brain tissue, postoperative inflammation, edema and fibrin deposits accompanying the transplantation of embryonic nerve tissue, create conditions for increasing the permeability of the blood-brain barrier with violation of autotolerance, sensitization and activation of CCD + CD4 + lymphocytes. The presentation of auto- and alloantigens is performed by astrocytes and microglial cells that react to y-INF expression of MHC molecules, ICAM-1, LFA-I, LFA-3, costimulatory molecules B7-1 (CD80) and B7-2 (CD86), and secretion of IL-la, IL-ip and y-INF.
Consequently, the fact of longer survival of embryonic nerve tissue in intracerebral transplantation than with its peripheral administration can hardly be attributed to the lack of initiation of transplantation immunity. Moreover, monocytes, activated lymphocytes (cytotoxic CD3 + CD8 + and T-cell helper cells) and the cytokines that they produce, as well as antibodies to the peripheral graft antigens of the embryonic neural tissue, play a major role in the process of its rejection. The low level of expression of MHC molecules in the embryonic neural tissue has a definite value in creating conditions for the longer stability of neurotransplants to T-cell immune processes. That is why in the experiment, immune inflammation after transplantation of embryonic neural tissue into the brain develops more slowly than after skin transplantation. Nevertheless, after 6 months, a complete destruction of individual grafts of the nervous tissue is observed. At the same time, T-lymphocytes restricted to MHC class II antigens are localized in the transplant zone (Nicholas et al., 1988). It has been experimentally established that with xenological neurotransplantation depletion of the level of T-helpers (L3T4 +), but not of cytotoxic T-lymphocytes (Lyt-2), prolongs the survival of rat nerve tissue in the brain of recipient mice. The rejection of the neurotransplant is accompanied by its infiltration by macrophages and host T-lymphocytes. Consequently, macrophages and activated host microglial cells act in situ as antigen-presenting immunostimulating cells, and increasing the expression of MHC class I donor antigens enhances the killer activity of the cytotoxic T-lymphocytes of the recipient.
It makes no sense to analyze numerous speculative attempts to explain the fact of neurotransplant rejection by the reaction of the immune system of the recipient organism to endotheliocytes or glial elements of the donor, as the pure lines of neural progenitor cells undergo an immune attack. It is worth mentioning that in the mechanisms of longer survival of the graft within the CNS, expression of the Fas-ligands, which bind the receptors of apoptosis (Fas-molecules) on T-lymphocytes infiltrating the brain and the apoptosis inducing them, plays an important role within the CNS, which is typical protective mechanism of barrier autoimmunogenic tissues.
As V. Tsymbalyuk and co-authors (2001) rightly noted, transplantation of embryonic neural tissue is characterized by the development of inflammation with the participation of brain and activated cells sensitized to antigens, antibodies, and also due to local production of cytokines. An important role in this is played by the pre-existing sensitization of the organism to brain antigens that occurs during the development of CNS diseases and can be directed to transplant antigens. That is why the really long-term survival of histone-compatible neurotransplants is achieved only by suppressing the immunity system with cyclosporin A or by administering monoclonal antibodies to the CD4 + lymphocytes of the recipient.
Thus, many problems of neurotransplantation remain unresolved, including those related to immunological compatibility of tissues, which can be resolved only after purposeful fundamental and clinical studies.