Stem cells and regenerative plastic medicine
Last reviewed: 23.04.2024
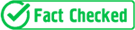
All iLive content is medically reviewed or fact checked to ensure as much factual accuracy as possible.
We have strict sourcing guidelines and only link to reputable media sites, academic research institutions and, whenever possible, medically peer reviewed studies. Note that the numbers in parentheses ([1], [2], etc.) are clickable links to these studies.
If you feel that any of our content is inaccurate, out-of-date, or otherwise questionable, please select it and press Ctrl + Enter.
Today, few practitioners are aware of the development of a new direction in the treatment of incurable diseases by traditional and non-traditional medicine. It's about regenerative-plastic medicine, based on the use of the regenerative potential of stem cells. Around the developing direction, an unprecedented scientific discussion arose and a pseudoscientific hullabaloo, largely created by the information hyperbole of the World Wide Web. In a very short time, laboratory studies of the therapeutic possibilities of stem cells went beyond the limits of the experiment and began to be actively introduced into practical medicine, which caused a lot of problems of scientific, ethical, religious, legal and legislative plan. State and public institutions were clearly not ready for the speed of the transition of stem cells from petri dishes to systems for intravenous administration, which does not benefit both society as a whole and a particular suffering person. In an unimaginable quantity and quality of information about the possibilities of stem cells, it is not easy to understand specialists (which in general is not, because everyone is trying to learn a new science independently), not to mention doctors who are not directly involved in regenerative plastic medicine.
Why do we need such experiments and do they need anything at all?
At first glance, the creation of cellular interspecific chimeras is the fruit of an unrestrained fantasy of a scientist-fanatic who has forgotten about bioethics. However, this approach significantly expanded our fundamental knowledge of embryogenesis, since it allowed us to count the number of cells necessary for organogenesis (formation of the liver, brain, skin, immune system organs). In addition (perhaps this is the main thing in the biology of ESC), geneticists have at their disposal a unique tool with the help of which the chimerization of embryos can establish the functional purpose of genes. First, the special technique of double knockout in the ESC is "turned off" the pair of genes under investigation. Then such ESCs are injected into the blastocyst and monitor the changes that occur in the body of the developing chimeric embryo. Thus, the functions of sf-1 genes (development of the adrenal and genital organs), urt-l (kidney bookmark), muD (development of skeletal muscles), gata-l-4 (laying of erythro- and lymphopoiesis) were established. In addition, in the ESC of laboratory animals, it is possible to introduce (transfected) human genes not yet studied to determine their function with the help of a chimeric embryo.
But, as a rule, the justification of the experiment by obtaining new fundamental knowledge does not meet the support of a wide audience. Let us give an example of an applied value of chimerization with the help of ESC. First of all, this is xenotransplantation, that is, transplanting the organs of an animal to humans. Theoretically, the creation of human-pig-cell cellular chimeras makes it possible to obtain an animal that is much closer in antigenic characteristics to an ESC donor, which in various clinical situations (diabetes mellitus, cirrhosis) can save a life for a sick person. True, for this you must first learn how to return the property of totipotency to the genome of a mature somatic cell, after which it can be introduced into the developing pig embryo.
Today, the property of ESC under special culture conditions is practically infinitely used to generate totipotent cell mass, with its subsequent differentiation into specialized cells, for example dopaminergic neurons, which are then transplanted to a patient with Parkinson's disease. In this case, transplantation is necessarily preceded by a directed differentiation of the resulting cell mass into the specialized cells needed for treatment and purification of the latter from undifferentiated cellular elements.
As it turned out later, the threat of carcinogenesis was not the only obstacle in the way of cell transplantation. Spontaneously, ESCs in the embryoid bodies are differentiated heterogeneously, that is, they form derivatives of the most diverse cell lines (neurons, keratinocytes, fibroblasts, endotheliocytes). In the field of view of the microscope, in this case, among the cells of various phenotypes, cardiomyocytes are distinguished, each of which contracts in its rhythm. However, for the treatment of a patient, it is necessary to have pure cell populations: neurons - with stroke, cardiomyocytes - with myocardial infarction, pancreatic β-cells - with diabetes mellitus, keratinocytes - with burns, etc.
The next stage in the development of cell transplantology was related to the development of technologies for obtaining a sufficient number (millions of cells) of such pure cell populations. The search for factors causing directional differentiation of ESCs was of an empirical nature, since the sequence of their synthesis during the process of embryogenesis remained unknown. First, it was found that the formation of the yolk sac is induced by the addition of cAMP and retinoic acid to the culture. Hematopoietic cell lines were formed by the presence in the culture medium of 1L-3, SCF, fibroblast growth factor (FGH), insulin-like growth factor (IGF-1), 1L-6 and granulocyte colony-stimulating factor (G-CSF). The cells of the nervous system were formed from the ESC after removal of LIF and a layer of fibroblasts acting as a feeder. After treatment with retinoic acid in the presence of embryonic serum, ESCs began to differentiate into neurons, and cardiomyocytes were prepared by the addition of dimethyl sulfoxide (DMSO), which provides targeted delivery of hydrophobic signaling molecules to the cell nucleus. In this case, the accumulation in the culture medium of active oxygen species, as well as electrical stimulation, contributed to the formation of mature contracting cardiomyocytes.
Huge forces and means were spent on the search for conditions for the differentiation of ESC into insulin-producing pancreatic cells. However, it soon became clear that a number of specialized cell lines of the pancreatic β-cells, immune and endocrine systems, adipocytes) do not arise from ESCs when stimulated by the principle of "one stimulating factor-one cell line". This principle turned out to be valid only for a limited number of cell lines. In particular, the formation of neurons can be induced by retinoic acid, muscle-cell cells by the transforming growth factor-β (TSP-β), erythroid lines - 1L-6, monocyte-myeloid line-1L-3. And the effects of these factors on the differentiation of ESC were strictly dose-dependent.
The stage of search for combinations of growth factors that would advance the ESC to later stages of embryogenesis with the formation of mesoderm (source of cardiomyocytes, skeletal muscles, epithelium of renal tubules, myeloerythropoiesis and smooth muscle cells), ectoderm (epidermis, neurons, retina) and endoderm (epithelium of the small intestine and secretory glands, pneumocytes). Nature, as it were, made the researchers move forward along the path of embryogenesis, repeating its steps in the Petri dish, without giving the possibility to get the desired result immediately and easily. And such combinations of growth factors have been found. Activin A in combination with TGF-β proved to be a potent stimulator of the formation of mesodermal cells from the ESC, while blocking the development of ento and ectoderm. Retinoic acid and also a combination of signals of bone marrow morphogenetic protein (BMP-4) and epidermal growth factor (EGF) activate the formation of ecto- and mesoderm cells, stopping the development of the endoderm. Intensive growth of cells of all three embryonic leaves is observed with the simultaneous effect on the ESC of two factors - the growth factor of hepatocytes (HGF) and the growth factor of nerve cells.
Thus, in order to obtain the desired cell lines, it is first necessary to transfer the embryonic stem cells to the stage of formation of the cells of any embryonic leaf, and then to select a new combination of growth factors capable of inducing directional differentiation of ecto-, meso- and endoderms into specialized cells necessary for transplantation patient. The number of combinations of growth factors for today is estimated in the thousands, most of them are patented, some are not disclosed at all by biotechnology firms.
It was the turn of the stage of purification of the obtained cells from undifferentiated cell impurities. The cells differentiated in culture were labeled with markers of mature cell lines and passed through a high-speed laser immunophenotypic sorter. The laser beam found them in a common cellular stream and directed along a separate path. The obtained purified cell material was first obtained by laboratory animals. It's time to evaluate the effectiveness of the use of ESK derivatives on models of diseases and pathological processes. One such model was experimental Parkinson's disease, which is well reproduced in animals with chemical compounds that destroy dopaminergic neurons. Because the underlying disease in humans is the acquired deficit of dopaminergic neurons, the use of replacement cell therapy in this case was pathogenetically justified. In animals with experimental hemiparkinsonism, about half of the dopaminergic neurons that were derived from the ESC and inserted into the brain structures survived. This was enough to significantly reduce the clinical manifestations of the disease. Attempts to restore the function of damaged CNS structures during experimental stroke, trauma and even spinal cord fractures were quite successful.
However, it should be noted that almost all cases of successful application of differentiated derivatives of ESC to correct experimental pathology were made in the acute period of the simulated pathological situation. The long-term results of treatment were not so comforting: after 8-16 months the positive effect of cell transplantation disappeared or sharply decreased. The reasons for this are quite understandable. Differentiation of transplanted cells in vitro or in loco morbi inevitably leads to the expression of cellular markers of genetic alienity, which provokes an immune attack from the recipient organism. To solve the problem of immunological incompatibility, traditional immunosuppression was used, in parallel to which clinical trials were begun to realize the potential of transdifferentiation and genetic correction of autologous hematopoietic and mesenchymal stem cells that do not cause immune conflict.
What is regenerative-plastic medicine?
Evolution has identified two basic options for the completion of cell life - necrosis and apoptosis, which at the tissue level are associated with proliferation and regeneration. Proliferation can be considered as a kind of sacrifice, when the filling of a defect in damaged tissue occurs due to its replacement by connective tissue elements: retaining structural integrity, the organism partially loses the function of the affected organ, which determines the subsequent development of compensatory reactions with hypertrophy or hyperplasia of structural and functional elements that have not been damaged. The length of the compensation period depends on the volume of structural lesions caused by the factors of primary and secondary alteration, after which in the vast majority of cases decompensation, sharp deterioration in quality and shortening of a person's life span begins. Physiological regeneration provides the processes of remodeling, that is, the replacement of the aging and dying by the mechanisms of natural cell death (apoptosis) of cells into new cells, stemming from the stem cell reserves of the human body. In the processes of reparative regeneration, the cellular resources of stem spaces are also involved, which, however, are mobilized in pathological conditions associated with disease or damage to tissues that initiate cell death through necrosis mechanisms.
The close attention of scientists, doctors, the press, television and the public to the problem of studying the biology of the embryonic stem cell (ESC) is primarily due to the high potential possibilities of cellular or, as we call it, regenerative-plastic therapy. The basis for the development of methods for treating severe human diseases (degenerative pathology of the central nervous system, spinal cord and brain injury, Alzheimer's and Parkinson's diseases, multiple sclerosis, myocardial infarction, arterial hypertension, diabetes mellitus, autoimmune diseases and leukemia, burn disease and neoplastic processes not a complete list of them) are laid unique properties of stem cells, which allow the creation of new tissues in return, as previously thought, irreversibly damaged tissue zones n sick body.
The progress of theoretical studies of stem cell biology over the past 10 years has been realized by spontaneously emerging trends in the emerging regenerative and plastic medicine, the methodology of which is not only fully systematized, but also needs it. The first and most rapidly developing field of practical use of the regenerative potential of stem cells has become replacement regenerative-plastic therapy. Its path is easily traced in the scientific literature - from experiments on animals with myocardial necrosis to recent work aimed at restoring the postinfarction deficit of cardiomyocytes or to replenishing the losses of β cells of the pancreas and dopaminergic neurons of the central nervous system.
Cell transplantation
The basis of replacement regenerative-plastic medicine is cell transplantation. The latter should be defined as a complex of medical measures in which, for a short or long time, the patient's organism has direct contact with viable cells of auto-, allo-, iso- or xenogeneic origin. The means of cell transplantation is a suspension of stem cells or their derivatives, standardized by the number of transplantation units. The transplantation unit is the ratio of the number of colony forming units in culture to the total number of transplanted cells. Methods of carrying out cellular transplantation: intravenous, intraperitoneal, subcutaneous injection of a suspension of stem cells or their derivatives; injection of a suspension of stem cells or their derivatives into the ventricles of the brain, lymphatic vessels or cerebrospinal fluid.
In allo- and autologous cell transplantation, two fundamentally different methodological approaches to the realization of the pluri-, multi- or pluripotential potential of stem cells - in vivo or in vitro - are used. In the first case, the introduction of stem cells into a diseased organism is carried out without their preliminary differentiation, in the second - after multiplication in culture, directed differentiation and purification from undifferentiated elements. Among the numerous methods of substitutive cell therapy, three groups of methods are quite clearly distinguished: replacement of bone marrow and blood cells, replacement of cells of organs and soft tissues, replacement of rigid and solid elements of the body (cartilage, bone, tendons, heart valves and vessels of capacitive type). The latter direction should be defined as reconstructive-regenerative medicine, since the potential of stem cell differentiation is realized on a matrix - a biologically inert or absorbable structure that has the form of a replaceable part of the body.
Another way to increase the intensity of regeneration and plastic processes in the affected tissues is the mobilization of the patient's own stem resources by using exogenous growth factors, such as granulocyte and granulocyte-macrophage colony-stimulating factors. In this case, the rupture of stromal connections leads to an increase in the yield of hematopoietic stem cells into the total bloodstream, which in the tissue damage zone provides regeneration processes due to their inherent plasticity.
Thus, the methods of regenerative medicine are aimed at stimulating the processes of restoring the lost function - either through the mobilization of its own stem reserves of the diseased organism, or by introducing allogenic cellular material.
An important practical result of the discovery of embryonic stem cells is therapeutic cloning, based on the understanding of the triggering mechanisms of embryogenesis. If the initial signal for the onset of embryogenesis is the pre-mRNA complex located in the cytoplasm of the oocyte, then the introduction of the nucleus of any somatic cell into the enucleated egg should trigger the embryo development program. Today we already know that about 15 000 genes take part in the implementation of the embryogenesis program. What happens to them afterwards, after birth, during periods of growth, maturity and aging? The answer to this question was given by Dolly the sheep: they are preserved. Using the most modern methods of research, it has been proved that the nuclei of adult cells store all the codes necessary for the formation of embryonic stem cells, embryonic leaflets, organogenesis and restriction maturation (differentiation and specialization) of mesenchymal, ecto-, endo- and mesodermal . Therapeutic cloning as a direction was formed already at the earliest stages of the development of cellular transplantology and provides for the return of totipotency to the patient's own somatic cells for obtaining a genetically identical transplant material.
The discovery of stem cells began "from the end", since the term introduced into biology and medicine by A. Maximov referred to bone marrow stem cells that give rise to all mature cellular elements of peripheral blood. However, hematopoietic stem cells, like cells of all tissues of an adult organism, also have a differentiated predecessor. A common source for absolutely all somatic cells is the embryonic stem cell. It should be noted that the concepts of "embryonic stem cells" and "embryonic stem cells" are by no means identical. Embryonic stem cells were isolated by J. Thomson from the internal cell mass of the blastocyst and transferred to long-lived cell lines. Only these cells have the facsimile "ESC". Leroy Stevens, who discovered embryonic stem cells in experiments in mice, called them "embryonic pluripotent stem cells," meaning the ability of ESC to differentiate into the derivatives of all three embryonic leaflets (ecto-, meso-, and endoderm). But all the cells of the embryo of later stages of development are also stem cells, since they give rise to a huge number of cells that form the body of an adult. To define them we propose the term "embryonic pluripotent progenitor cells".
[4], [5], [6], [7], [8], [9], [10], [11]
Types of stem cells
The modern classification of stem cells is based on the principle of their separation according to their ability (potency) to give rise to cellular lines, which is defined as totality, pluri-, multi-, poly-, bi-and unipotency. Totipotency, that is, the ability to recreate a genetically programmed organism as a whole, has zygotic cells, blastomeres and embryonic stem cells (blastocyst inner mass cells). Another group of totipotent cells, which are formed at later terms of embryo development, is represented by primary hermetic cells of the embryonic sexual zone (genital tubercles). Pluripotency, under which the ability to differentiate into cells of any organ or tissue, is inherent in the embryonic cells of three germinal leaves - ecto-, meso-, and endoderm. It is believed that multipotency, that is, the ability to form any cells within a single specialized line, is characteristic of only two types of cells: the so-called mesenchymal stem cells that are formed in the neural crest and are the precursors of all cells of the connective tissue base of the organism, including neuroglia cells, as well as hematopoietic hematopoietic stem cells, which gives rise to all lines of blood cells. In addition, bi-and unipotent stem cells are isolated, in particular, the progenitor cells of the myeloid, lymphoid, monocytic and megakaryocytic hematopoietic sprouts. The existence of unipotent stem cells is clearly demonstrated on the example of liver cells - the loss of a significant part of the hepatic tissue is compensated for by the intensive division of differentiated polyploid hepatocytes.
In the process of development, all organs and tissues are formed as a result of the proliferation and differentiation of the internal cell mass of the blastocyst, the cells of which are in the strict sense totipotent embryonic stem cells. The first work on isolating embryonic stem cells was performed by Evans, who showed that blastocysts implanted in the brains of mice give rise to teratocarcinomas whose cells clone form lines of pluripotent embryonic stem cells (the initial name for these cells is embryonal carcinoma cells or in the abbreviation ECC in currently not applicable). These data were confirmed in a number of other studies in which embryonic stem cells were obtained by culturing blastocyst cells of mice and other animals as well as humans.
In the literature of recent years, there are more and more reports on the plasticity of stem cells, which are considered not only as the ability of the latter to differentiate into different types of cells at different stages of development, but also to undergo dedifferentiation (transdifferentiation, retrodifferentiation). That is, it is possible in principle to return the somatic differentiated cell to the stage of embryonic development with recapitulation (return) of pluripotency and its realization into a differentiation with the formation of cells of a different type. It is reported, in particular, that hematopoietic stem cells are capable of transdifferentiating with the formation of hepatocytes, cardiomyoblasts and endotheliocytes.
The scientific debate regarding the separation of stem cells through their plasticity continues, that is, the terminology and glossary of cell transplantation are in the process of becoming, which is of direct practical importance, since it is on the use of plastic properties and the ability of stem cells to differentiate into different cell lines that most methods of regenerative plastic medicine.
The number of publications in the field of fundamental and applied problems of regenerative and plastic medicine is growing rapidly. The range of various methodological approaches aimed at the most optimal use of the regenerative-plastic potential of stem cells has already been outlined. Zones of their vital interests were defined by cardiologists and endocrinologists, neuropathologists and neurosurgeons, transplantologists and hematologists. In the plastic possibilities of stem cells, ophthalmologists, tuberculosis specialists, pulmonologists, nephrologists, oncologists, genetics, pediatricians, gastroenterologists, therapists and pediatricians, surgeons and obstetrician-gynecologists are looking for solutions to acute problems. All representatives of modern medicine hope to get a cure for the fatal diseases that have been considered so far.
Is cell transplantation another "panacea" from all ills?
This question arises quite rightly among all doctors and scientists who are thoughtful and analyzing the current state of medical science. The situation is complicated by the fact that on one side of the field of scientific confrontation there are "healthy conservatives", on the other - "sick fanatics" of cell transplantology. Obviously, the truth, as always, lies between them - on a "no-man's land". Without touching on issues of law, ethics, religion and morality, let us consider the pros and cons of the indicated areas of regenerative and plastic medicine. The "light breeze" of the first scientific reports on the therapeutic possibilities of ESCs already a year after their discovery turned into a "gust of wind", swirling in 2003 in the "information tornado." The first series of publications concerned the cultivation of embryonic stem cells, their multiplication and directed differentiation in vitro.
It turned out that for unlimited reproduction of embryonic stem cells in culture, a number of conditions must be strictly observed. Three factors must necessarily be present in the conditioned environment: interleukin-6 (IL-6), stem cell factor (SCF) and leukosinhibiting factor (LIF). In addition, embryonic stem cells should be grown on a substrate (a feeder layer of cells) from embryonic fibroblasts and in the presence of fetal calf serum. Under these conditions, ESCs in the culture grow clones and form embryoid bodies - aggregates of suspension clones of globular cells. The most important feature of the ESC clone is that in the culture the embryoid body ceases to grow when accumulated in the aggregate 50-60, a maximum of 100 cells. During this period, an equilibrium state sets in - the rate of cell division within the clone is equal to the rate of apoptosis (programmed cell death) at its periphery. After reaching such a dynamic equilibrium, the peripheral cells of the embryoid body undergo spontaneous differentiation (usually with the formation of endodermal yolk sac, angioblast and endotheliocyte fragments) with loss of totipotency. Therefore, to obtain a sufficient amount of totipotent cell mass, the embryonic body needs to be disaggregated weekly with a transplantation of individual embryonic stem cells into a new nutrient medium, a process that is laborious enough.
The discovery of embryonic stem cells did not give an answer to the question of what exactly and how it launches embryogenesis programs encoded in the zygote DNA. It remains unclear how the program of the genome unfolds in the process of human life. At the same time, the study of embryonic stem cells made it possible to develop a concept about the mechanisms for preserving the totality, pluri- and multipotency of stem cells in the process of their division. The main distinguishing feature of the stem cell is its ability to self-reproduction. This means that the stem cell, unlike the differentiated one, is divided asymmetrically: one of the daughter cells gives rise to a specialized cell line, and the second preserves the totality, pluri- or multipotency of the genome. It remained unclear why and how this process occurs at the earliest stages of embryogenesis, when the dividing internal cellular mass of blastocysts is all totipotent, and the ESC gene is in a dormant (dormant, inhibited) state. If the process of duplication necessarily precedes the activation and expression of a whole complex of genes when dividing an ordinary cell, then this does not happen when dividing the ESC. The answer to the question "why" was obtained after the discovery of pre-existing mRNA (pre-mRNA) in the ESC, some of which are formed in follicular cells and remain in the cytoplasm of the ovum and the zygote. The second discovery answered the question "how": special enzymes, called "editases", were found in the ESC. Edithases perform three major functions. First, they provide an alternative epigenetic (without the involvement of the genome) reading and duplication of pre-mRNA. Secondly, they realize the process of activation of pre-mRNA (splicing - excision of introns, that is, inactive regions of RNA, which inhibit the process of protein synthesis on mRNA), after which the assembly of protein molecules begins in the cell. Thirdly, editases promote the formation of secondary mRNA, which are the repressors of the mechanisms of gene expression, which preserves the dense packing of chromatin and the inactive state of the genes. Protein products synthesized on such secondary mRNAs and called protein-silencer or genomic guardians are present in human ovules.
This is how the mechanism for the formation of immortal cell lines of embryonic stem cells is represented today. Simply put, the signal to start the embryogenesis program, whose initial stages consist in the formation of totipotent cell mass, comes from the cytoplasm of the egg cell. If at this stage the internal cellular mass of the blastocyst, that is, the ESC, is isolated from further regulatory signals, the process of cell self-reproduction takes place in a closed cycle without the involvement of the genes of the cell nucleus (epigenetically). If we provide such a cell with nutrient material and isolate it from external signals that promote the differentiation of the cell mass, it will divide and reproduce itself similar infinitely.
The first results of experimental attempts to use totipotent cells for transplantation were very impressive: the introduction of embryonic stem cells into tissues of mice with weakened immunosuppressors by the immune system in 100% of cases led to the development of tumors. Among the neoplasmic cells from which the ESC originated, differentiated derivatives of totipotent exogenous cell material, in particular neurons, were found, but the growth of teratocarcinoma reduced the value of the results obtained to naught. At the same time, in the works of L. Stevens, ESCs, introduced into the abdominal cavity, formed large aggregates in which embryonic muscles, heart, hair, skin, bones, muscles and nervous tissue were fragmentarily formed. (Surgeons who opened dermoid cysts, this picture should be familiar). It is interesting that suspended mouse embryoblast cells behave in exactly the same way: their introduction into adult tissues of immunocompromised animals always causes the formation of teratocarcinomas. But if a clear line of ESC is isolated from this tumor and inserted into the abdominal cavity, then again, specialized somatic derivatives of all three embryonic sheets without signs of carcinogenesis are formed.
Thus, the next problem that needed to be solved was the purification of the cellular material from the impurities of undifferentiated cells. However, even with very high efficiency of directed cell differentiation, up to 20% of the cells in the culture retain their totipotent potential, which in vivo, unfortunately, is realized in tumor growth. Another "slingshot" of nature - on the scales of the scales of medical risk the guarantee of recovery of the patient balances with the guarantee of his death.
The relationship between tumor cells and the more advanced in development than embryonic pluripotent progenitor cells (EECC) is very ambiguous. The results of our studies have shown that the administration of ESRP to various transplanted tumors in rats can lead to the disintegration of tumor tissue (D), a rapid increase in tumor mass (D), its reduction (E-3), or does not affect the size of spontaneous central focal necrosis Neoplastic tissue (I, K). It is obvious that the result of the interaction of EKPK and tumor cells is determined by the total set of cytokines and growth factors produced by them in vivo.
It is noteworthy that the embryonic stem cell, responding to carcinogenesis for contact with the tissues of an adult organism, is perfectly assimilated with the cell mass of the embryo, integrating into all the organs of the embryo. Such chimeras, consisting of intrinsic embryonic cells and donor ESCs, are called allophenic animals, although, in fact, they are not phenotypic chimeras. Maximum cell chimerization when introducing ESC into the early embryo undergoes a hematopoietic system, skin, nerve tissue, liver and small intestine. Cases of chimerization of genital organs are described. The only untouchable zone for the ESA was the primary sex cells.
That is, the embryo stores the genetic information of its parents, which preserves the purity and continuation of both the genus and the species.
In the conditions of blocking the division of cells of the early embryo with the help of cytoclasin, the introduction of embryonic stem cells into the blastocyst leads to the development of the embryo, in which primary germ cells, like all others, were formed from donor embryonic stem cells. But in this case, the embryo itself is completely donor, genetically alien to the organism of the surrogate mother. The mechanisms of such a natural block of the potential possibility of mixing own and foreign hereditary information have not yet been clarified. It can be assumed that in this case an apoptosis program is implemented, the determinants of which are not yet known.
Attention should be paid to the fact that embryogenesis of animals of different species is never coordinated: when the donor organogenesis program is implemented in the body of the embryo-recipient of xenogeneic embryonic stem cells, the embryo dies in utero and resorbed. Therefore, the existence of rat-mouse, pig-cow, and rat-man chimeras must be understood as cellular, but not morphological mosaicism.In other words, when a single mammal is introduced into a blastocyst of another species, the progeny of the maternal species always develops, in which among the own cells of almost all organs there are inclusions and sometimes clusters of structural and functional units consisting of genetically alien material of ESK derivatives. "The term" humanized naya pig "as a designation of a certain monster endowed with reason or external signs of a person. This is just an animal, part of the cells of the body of which comes from the pigs of the human ESCs injected into the blastocyst.
The prospect of using stem cells
It has long been known that diseases associated with the genopathology of the cells of the hematopoietic and lymphoid lines are often eliminated after allogeneic bone marrow transplantation. The replacement of one's own hematopoietic tissue with genetically normal cells of a related donor leads to partial and sometimes complete recovery of the patient. Among the genetic diseases that are treated with the allogeneic bone marrow transplantation, the syndrome of combined immunodeficiency, X-linked agammaglobulinemia, chronic granulomatosis, Wiskott-Aldrich syndrome, Gaucher and Harler diseases, adrenoleukodystrophy, metachromatic leukodystrophy, sickle cell anemia, thalassemia, anemia Fanconi and AIDS. The main problem in the use of allogeneic bone marrow transplantation in the treatment of these diseases is associated with the selection of an HbA-compatible related donor, for the successful search of which, on average, 100,000 samples of a typical donor hemopoietic tissue are required.
Gene therapy allows you to correct a genetic defect directly in the stem hemopoietic cells of the patient. Theoretically, gene therapy offers the same advantages in the treatment of genetic diseases of the hematopoiesis system, as allogeneic bone marrow transplantation, but without all possible immunological complications. However, this requires a technique that allows efficient transfer of a complete gene into stem hemopoietic cells and maintains the necessary level of its expression, which may not be very high in certain types of hereditary pathology. In this case, even an insignificant replenishment of the protein product of a deficient gene produces a positive clinical effect. In particular, with hemophilia B, 10-20% of the normal level of factor IX is sufficient to restore the internal mechanism of blood coagulation. Genetic modification of autologous cellular material was successful in experimental hemiparkinsonism (unilateral destruction of dopaminergic neurons). Transfection of rat embryonic fibroblasts with a retroviral vector containing the tyrosine hydroxylase gene ensured the synthesis of dopamine in the central nervous system: intracerebral administration of transfected fibroblasts sharply reduced the intensity of clinical manifestations of the experimental model of Parkinson's disease in experimental animals.
The prospect of using stem cells for gene therapy of human diseases has posed many new challenges for clinicians and experimenters. The problematic aspects of gene therapy are associated with the development of a safe and efficient system for transporting the gene into the target cell. At the moment, the efficiency of gene transfer into cells of large mammals is very small (1%). Methodically, this problem is solved in various ways. Gene transfer in vitro is the transfection of genetic material into the patient's cells in culture, and then returning them to the patient's body. This approach should be considered optimal when using genes introduced into bone marrow stem cells, since the methods of transferring the hematopoietic cells from the organism to the culture and back are well developed. Most often for the transfer of the gene into the hematopoietic cells in vitro, retrovi rus are used. However, the bulk of stem hemopoietic cells is at rest, which makes it difficult to transport genetic information with the help of retroviruses and requires the search for new ways of efficient gene transfer into the dermal stem cells. Currently, gene transfer methods such as transfection, direct microinjection of DNA into cells, lipofection, electroporation, "gene gun", mechanical connection with glass beads, transfection of hepatocytes with a receptor-dependent DNA compound with asialoglycoprotein, as well as aerosol introduction of a transgene into cells of the alveolar epithelium of the lungs. The efficiency of DNA transfer by these methods is 10.0-0.01%. Other words, depending on the method of introduction of genetic information, success can be expected in 10 patients out of 100 or in 1 patient out of 10 LLC patients. Obviously, an effective and at the same time safe method of transferring therapeutic genes has yet to be developed.
A fundamentally different solution to the problem of rejection of allogeneic cellular material in cell transplantology is the use of high doses of embryonic pluripotent progenitor cells to achieve the effect of reinstallation of the control system of the antigenic homeostasis of an adult organism (the effect of Kuharchuk-Radchenko-Sirman), whose essence consists in inducing immunological tolerance by creating a new base of immunocompetent cells while reprogramming the antigenic home control system stasis. After the administration of large doses of EPPK, the latter are fixed in the tissues of the thymus and bone marrow. In the thymus, EPPK under the influence of a specific microenvironment differentiate into dendritic, interdigital cells and epithelial-stromal elements. In the course of differentiation of EKPK in the thymus of the recipient, along with the own molecules of the main histocompatibility complex (MHC), MHC molecules are expressed which are genetically determined in donor cells, that is, a double standard of MHC molecules is established, according to which positive and negative selection of T lymphocytes is realized.
Thus, the renewal of the effector link of the immune system of the recipient organism occurs according to the known mechanisms of positive and negative selection of T lymphocytes, but through a double standard of MHC receptor molecules and donor EDCMs.
Reprogramming the immune system with EKPK not only allows carrying out cellular transplantation without subsequent prolonged use of immunosuppressants, but also opens up entirely new prospects in the treatment of autoimmune diseases, and provides a fulcrum for the development of new ideas about the process of human aging. To understand the mechanisms of aging, we proposed a theory of depletion of the body's stem spaces. According to the basic position of this theory, aging is a permanent reduction in the size of the body's stem spaces, by which is meant the pool of regional ("adult") stem cells (mesenchymal, neuronal, hematopoietic stem cells, progenitor cells of the skin, digestive tract, endocrine epithelium, pigment cells of ciliary folds, etc.), replenishing the cell loss of the corresponding tissue in the process of body remodeling. Remodeling of an organism is an update of the cellular composition of all tissues and organs at the expense of cells of stem spaces, which continues throughout the life of a multicellular organism. The number of cells in the stem spaces is determined genetically, which determines the limited size (proliferative potential) of each stem space. In turn, the dimensions of stem spaces determine the aging rate of individual organs, tissues and body systems. After the depletion of the cellular reservoirs of stem spaces, the intensity and rate of aging of the multicellular organism is determined by the mechanisms of aging of somatic differentiated cells within the Hayflick limit.
Consequently, at the stage of postnatal ontogeny, the expansion of stem spaces can not only significantly increase the duration, but also improve the quality of life by restoring the body's remodeling potential. Extension of stem spaces can be achieved by administering large doses of allogeneic embryonic pluripotent progenitor cells provided that the immune system of the recipient is simultaneously reprogrammed, which in the experiment substantially increases the life span of the old mice.
The theory of depletion of stem spaces can change existing concepts not only about the mechanisms of aging, but also about the disease, as well as the consequences of its medical-cytotoxic treatment. In particular, the disease can develop as a result of the pathology of cells in stem spaces (oncopathology). The depletion of the reserve of mesenchymal stem cells disrupts the processes of remodeling of connective tissue, which leads to the appearance of external signs of old age (wrinkles, flabbiness of the skin, cellulite). Depletion of the stem reserve of endothelial cells causes the development of arterial hypertension and atherosclerosis. Initially, the small size of the thymus stem space determines its early permanent age involution. Premature aging is a consequence of the initial pathological reduction in the size of all the stem spaces of the body. Drug and non-pharmacological stimulation of stem cell reserves improves the quality of life by reducing its duration, as it reduces the size of stem spaces. The low effectiveness of modern geroprotectors is due to their protective effect on the aging differentiated somatic cell, and not on the stem spaces of the body.
In conclusion, once again, we note that regenerative-plastic medicine is a new direction in the treatment of human diseases, based on the use of the regenerative-plastic potential of stem cells. In this case, plasticity means the ability of exogenous or endogenous stem cells to be implanted and give rise to new specialized cell sprouts in damaged tissue areas of the diseased organism. The object of regenerative and plastic medicine is the incurable to this day fatal diseases of the person, hereditary pathology, diseases in which the traditional medicine methods achieve only a symptomatic effect, as well as anatomical defects of the body, the restoration of which is directed to reconstructive plastic regenerative surgery. The first attempts to recreate whole and at the same time functionally full-fledged organs from stem cells, in our opinion, it is too early to make a separate area of practical medicine. The subject of regenerative and plastic medicine are stem cells, which, depending on the source of their production, have a different regenerative-plastic potential. The methodology of regenerative-plastic medicine is based on the transplantation of stem cells or their derivatives.