Medical expert of the article
New publications
Protein metabolism: proteins and the need for them
Last reviewed: 23.04.2024
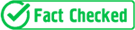
All iLive content is medically reviewed or fact checked to ensure as much factual accuracy as possible.
We have strict sourcing guidelines and only link to reputable media sites, academic research institutions and, whenever possible, medically peer reviewed studies. Note that the numbers in parentheses ([1], [2], etc.) are clickable links to these studies.
If you feel that any of our content is inaccurate, out-of-date, or otherwise questionable, please select it and press Ctrl + Enter.
Protein is one of the main and vitally important products. Now it has become obvious that the use of protein for energy costs is irrational, because as a result of the breakdown of amino acids, many acidic radicals and ammonia are formed that are not indifferent to the child's body.
What is protein?
There is no protein in the human body. Only with the breakdown of tissues, proteins split into them with the release of amino acids, which go to maintain the protein composition of other, more vital tissues and cells. Therefore, normal growth of the body without sufficient protein is impossible, since fats and carbohydrates can not replace them. In addition, proteins contain essential amino acids, necessary for building newly formed tissues or for their self-renewal. Proteins are an integral part of various enzymes (digestive, tissue, etc.), hormones, hemoglobin, antibodies. It is estimated that about 2% of muscle proteins are enzymes that are constantly updated. Proteins play the role of buffers, participating in maintaining a constant reaction of the environment in various fluids (blood plasma, spinal fluid, intestinal secrets, etc.). Finally, proteins are a source of energy: 1 g of protein, when it completely disintegrates, forms 16.7 kJ (4 kcal).
For the study of protein metabolism, a nitrogen balance criterion has been used for many years. To do this, determine the amount of nitrogen coming from the food, and the amount of nitrogen that is lost with the fecal masses and is excreted in the urine. On the loss of nitrogenous substances with feces, the degree of protein digestion and its resorption in the small intestine is judged. By the difference between food nitrogen and its release with feces and urine, it is judged the extent of its consumption for the formation of new tissues or their self-renewal. In children immediately after birth, or small and immature, the imperfection of the system of assimilation of any food protein, especially if it is not a protein of mother's milk, can lead to the impossibility of nitrogen utilization.
The timing of the formation of the functions of the gastrointestinal tract
Age, month |
FAO / WHO (1985) |
United Nations (1996) |
0-1 |
124 |
107 |
1-2 |
116 |
109 |
2-3 |
109 |
111 |
3 ^ |
103 |
101 |
4-10 |
95-99 |
100 |
10-12 |
100-104 |
109 |
12-24 |
105 |
90 |
In an adult, as a rule, the amount of nitrogen excreted is usually equal to the amount of nitrogen supplied with food. In contrast, children have a positive nitrogen balance, i.e., the amount of nitrogen fed with food always exceeds its loss with feces and urine.
The retention of nutrient nitrogen, and therefore its utilization by the body, depends on the age. Although the ability to retention of nitrogen from food persists throughout life, but it is greatest in children. The level of nitrogen retention corresponds to the growth rate and the rate of protein synthesis.
The rate of protein synthesis in different age periods
Age periods |
Age |
Synthetic rate, g / (kg • day) |
Newborn with low body weight |
1-45 days |
17.46 |
The child of the second year of life |
10-20 months |
6.9 |
Adult |
20-23 years |
3.0 |
Old man |
69-91 year |
1.9 |
The properties of food proteins, taken into account in the normalization of nutrition
Bioavailability (absorption):
- 100 (Npost - Nout) / Npost,
Where Npost is nitrogen supplied; Nvd - nitrogen, isolated with feces.
Net recovery (NPU%):
- (Npt-100 (Ncn + Nvh)) / Nin,
Where Ninj is the nitrogen of the food;
Nst - feces nitrogen;
Nmh is the urine nitrogen.
Coefficient of protein efficiency:
- Addition in body weight per 1 g of eaten protein in a standardized experiment on rats.
Amino acid "fast":
- 100 Akb / Ake,
Where Akb - the content of a given amino acid in a given protein, mg;
Ake - the content of this amino acid in the reference protein, mg.
As an illustration of the concept of "fast" and the concept of "ideal protein" we give data on the characteristics of the "fast" and the utilization of several food proteins.
Indicators of the "amino acid speed" and "clean utilization" of some food proteins
Protein |
Skor |
Recycling |
Maize |
49 |
36 |
Millet |
63 |
43 |
Rice |
67 |
63 |
Wheat |
53 |
40 |
Soybean |
74 |
67 |
Whole egg |
100 |
87 |
Women's milk |
100 |
94 |
Cow's milk |
95 |
81 |
Recommended protein intake
Considering the essential differences in the composition and nutritional value of proteins, calculations of protein supply at an early age produce only and exclusively proteins of the highest biological value, quite comparable in nutritional value with the protein of human milk. This also applies to the recommendations given below (WHO and M3 of Russia). In the older age groups, where the total protein requirement is somewhat lower, and with regard to adults, the problem of protein quality is satisfactorily solved when enriching the diet with several types of plant proteins. In intestinal chyme, where amino acids of various proteins and serum albumins are mixed, an amino acid ratio close to the optimal one is formed. The problem of protein quality is very acute when eating almost exclusively one kind of vegetable protein.
The general rationing of protein in Russia is somewhat different from sanitary regulation abroad and in WHO committees. This is due to some differences in the criteria for optimal provision. Over the years, there has been a convergence of these positions and various scientific schools. The differences are illustrated by the following tables of recommendations adopted in Russia and in WHO scientific committees.
Recommended protein intake for children under 10 years of age
Index |
0-2 months |
3-5 months |
6-11 months |
1-3 years |
3-7 years |
7-10 years old |
Whole proteins, g |
- |
- |
- |
53 |
68 |
79 |
Proteins, g / kg |
2.2 |
2.6 |
2.9 |
- |
- |
- |
Safe levels of protein intake in young children, g / (kg • day)
Age, month |
FAO / WHO (1985) |
United Nations (1996) |
0-1 |
- |
2.69 |
1-2 |
2.64 |
2.04 |
2-3 |
2.12 |
1.53 |
3 ^ |
1.71 |
1.37 |
4-5 |
1.55 |
1.25 |
5-6 |
1.51 |
1.19 |
6-9 |
1.49 |
1.09 |
9-12 |
1.48 |
1.02 |
12-18 |
1.26 |
1.00 |
18-24 |
1.17 |
0.94 |
Taking into account the different biological value of plant and animal proteins, it is customary to carry out the rationing both in terms of the amount of protein used, and the animal protein or its fraction in the total amount of protein consumed per day. An example is the table on the rationing of the M3 protein of Russia (1991) for children of older age groups.
The ratio of vegetable and animal protein in the recommendations for consumption
Proteins |
11-13 years old |
14-17 years old |
||
Boys |
Girls |
Boys |
Girls |
|
Whole proteins, g |
93 |
85 |
100 |
90 |
Including animals |
56 |
51 |
60 |
54 |
The Joint FAO / WHO Expert Group (1971) believes that a safe level of protein intake, calculated as cow milk protein or egg protein, is 0.57 g per kg of body weight per day for an adult male and 0.52 g / kg per woman per day. A safe level is the amount needed to meet physiological needs and maintain the health of almost all members of this population group. For children, the safe level of protein intake is higher than that of adults. This is due to the fact that in children the self-renewal of tissues takes place more vigorously.
It has been established that the assimilation of nitrogen by an organism depends on both the quantity and the quality of the protein. Under the latter, it is more correct to understand the amino acid composition of the protein, especially the presence of essential amino acids. The need of children in both protein and amino acids is much higher than that of an adult. It is estimated that a child needs about 6 times more amino acids than an adult.
The requirements for essential amino acids (mg per 1 g protein)
Amino acids |
Children |
Adults |
||
Up to 2 years |
2-5 years |
10-12 years old |
||
Histidine |
26th |
19 |
19 |
16 |
Isoleucine |
46 |
28 |
28 |
13 |
Leucine |
93 |
66 |
44 |
19 |
Lysine |
66 |
58 |
44 |
16 |
Methionine + cystine |
42 |
25 |
22 |
17th |
Phenylalanine + tyrosine |
72 |
63 |
22 |
19 |
Threonine |
43 |
34 |
28 |
9 |
Tryptophan |
17th |
Eleven |
9 |
5 |
Valine |
55 |
35 |
25 |
13 |
It can be seen from the table that the need for children in amino acids is not only higher, but the ratio of the need for vital amino acids is different for them than for adults. There are also different concentrations of free amino acids in plasma and in whole blood.
Especially great is the need for leucine, phenylalanine, lysine, valine, threonine. If we take into account that 8 amino acids (leucine, isoleucine, lysine, methionine, phenylalanine, threonine, tryptophan and valine) are vital for an adult, in children under 5 years of age, histidine is also an essential amino acid. In children of the first 3 months of life cystine, arginine, taurine are added to them, and in the premature still glycine, i.e., 13 amino acids for them are vitally important. This must be taken into account when building children's nutrition, especially the early age. Only due to the gradual maturation of enzyme systems in the process of growth, the need for children in essential amino acids gradually decreases. At the same time, with excessive protein overload in children is easier than in adults, there are amino acidemia, which can manifest as a delay in development, especially neuropsychiatric.
The concentration of free amino acids in the blood plasma and whole blood of children and adults, mol / l
Amino acids |
Blood plasma |
Whole blood |
||
Newborns |
Adults |
Children 1-3 years old |
Adults |
|
Alanin |
0.236-0.410 |
0.282-0.620 |
0.34-0.54 |
0.26-0.40 |
A-Aminobutyric acid |
0.006-0.029 |
0.008-0.035 |
0.02-0.039 |
0.02-0.03 |
Arginine |
0.022-0.88 |
0.094-0.131 |
0.05-0.08 |
0.06-0.14 |
Asparagine |
0.006-0.033 |
0.030-0.069 |
- |
- |
Aspartic acid |
0.00-0.016 |
0.005-0.022 |
0.08-0.15 |
0.004-0.02 |
Valine |
0.080-0.246 |
0.165-0.315 |
0.17-0.26 |
0.20-0.28 |
Histidine |
0.049-0.114 |
0.053-0.167 |
0.07-0.11 |
0.08-0.10 |
Glycine |
0.224-0.514 |
0.189-0.372 |
0.13-0.27 |
0.24-0.29 |
Glutamine |
0.486-0.806 |
0.527 |
- |
- |
Glutamic acid |
0.020-0.107 |
0.037-0.168 |
0.07-0.10 |
0.04-0.09 |
Isoleucine |
0.027-0.053 |
0.053-0.110 |
0.06-0.12 |
0.05-0.07 |
Leucine |
0.047-0.109 |
0.101-0.182 |
0.12-0.22 |
0.09-0.13 |
Lysine |
0,144-0,269 |
0.166-0.337 |
0.10-0.16 |
0.14-0.17 |
Methionine |
0.009-0.041 |
0.009-0.049 |
0.02-0.04 |
0.01-0.05 |
Ornithine |
0.049-0.151 |
0.053-0.098 |
0.04-0.06 |
0.05-0.09 |
Proline |
0.107-0.277 |
0,119-0,484 |
0.13-0.26 |
0.16-0.23 |
Serin |
0.094-0.234 |
0.065-0.193 |
0.12-0.21 |
0.11-0.30 |
Taurine |
0.074-0.216 |
0.032-0.143 |
0.07-0.14 |
0.06-0.10 |
Tyrosine |
0.088-0.204 |
0.032-0.149 |
0.08-0.13 |
0.04-0.05 |
Threonine |
0.114-0.335 |
0.072-0.240 |
0.10-0.14 |
0.11-0.17 |
Tryptophan |
0.00-0.067 |
0.025-0.073 |
- |
- |
Phenylalanine |
0.073-0.206 |
0.053-0.082 |
0.06-0.10 |
0.05-0.06 |
Cystine |
0.036-0.084 |
0.058-0.059 |
0.04-0.06 |
0.01-0.06 |
Children are more sensitive to starvation than adults. In countries where there is a sharp protein deficit in children's nutrition, mortality at an early age is 8-20 times higher. Since the protein is also necessary for the synthesis of antibodies, then, as a rule, when it is deficient in nutrition in children, there are often various infections that, in turn, increase the need for protein. A vicious circle is being created. In recent years, it has been established that protein deficiency in the diet of children of the first 3 years of life, especially prolonged, can cause irreversible changes that persist for life.
A number of indicators are used to judge protein metabolism. Thus, the determination in the blood (plasma) of the protein content and its fractions is a summary expression of the processes of protein synthesis and decomposition.
The content of total protein and its fractions (in g / l) in serum
Index |
The mother |
Blood of the |
In children aged |
||||
0-14 days |
2-4 weeks |
5-9 weeks |
9 weeks - 6 months |
6-15 months |
|||
Total protein |
59.31 |
54.81 |
51.3 |
50.78 |
53.37 |
56.5 |
60.56 |
Albumins |
27.46 |
32.16 |
30.06 |
29.71 |
35.1 |
35.02 |
36.09 |
α1-globulin |
3.97 |
2.31 |
2.33 |
2.59 |
2.6 |
2.01 |
2.19 |
α1-lipoprotein |
2.36 |
0.28 |
0.65 |
0.4 |
0.33 |
0.61 |
0.89 |
α2-globulin |
7.30 |
4.55 |
4.89 |
4.86 |
5.13 |
6.78 |
7.55 |
α2-Macroglobulin |
4.33 |
4.54 |
5.17 |
4.55 |
3.46 |
5.44 |
5.60 |
α2-haptoglobin |
1.44 |
0.26 |
0,15 |
0.41 |
0.25 |
0.73 |
1.17 |
α2-ceruloplasmin |
0.89 |
0.11 |
0.17 |
0.2 |
0.24 |
0.25 |
0.39 |
β-globulin |
10.85 |
4.66 |
4.32 |
5.01 |
5.25 |
6.75 |
7.81 |
β2-lipoprotein |
4.89 |
1.16 |
2.5 |
1.38 |
1.42 |
2.36 |
3.26 |
β1-siderophilin |
4.8 |
3.33 |
2.7 |
2.74 |
3.03 |
3.59 |
3.94 |
β2-A-globulin, ED |
42 |
1 |
1 |
3.7 |
18 |
19.9 |
27.6 |
β2-M-globulin, UE |
10.7 |
1 |
2.50 |
3.0 |
2.9 |
3.9 |
6.2 |
γ-Globulin |
10.9 |
12.50 |
9.90 |
9.5 |
6.3 |
5.8 |
7.5 |
The norms of protein and amino acids in the body
As can be seen from the table, the total protein content in the blood serum of a newborn is lower than that of its mother, which is explained by active synthesis, rather than by simple filtration of protein molecules through the placenta from the mother. During the first year of life, there is a decrease in the total protein content in the blood serum. Especially low rates in children aged 2-6 weeks, and starting from 6 months there is a gradual increase in it. However, at a younger school-age, the protein content is somewhat lower than the average in adults, and these deviations are more pronounced in boys.
Along with the lower content of total protein, there is a lower content of some of its fractions. It is known that the synthesis of albumins occurring in the liver is 0.4 g / (kg-day). In normal synthesis and elimination (albumin partially enters the intestinal lumen and is recycled, a small amount of albumin is excreted in the urine), the serum albumin content determined by electrophoresis is about 60% of the serum proteins. In a newborn, the percentage of albumin is even relatively higher (about 58%) than that of its mother (54%). This is explained, obviously, not only by the synthesis of albumin by the fetus, but also by the partial transplacental transition from the mother. Then, in the first year of life, the content of albumin decreases, going in parallel with the content of the total protein. The dynamics of the γ-globulin content is similar to that of albumin. Especially low indices of γ-globulins are observed during the first half of life.
This is explained by the disintegration of γ-globulins transplacentally derived from the mother (mainly immunoglobulins belonging to β-globulin).
Synthesis of their own globulins matures gradually, which is explained by their slow growth with age of the child. The content of α1, α2- and β-globulins is relatively little different from that of adults.
The main function of albumins is nourishing-plastic. Due to the low molecular weight of albumins (less than 60 000), they have a significant effect on the colloid-osmotic pressure. Albumins play an important role in the transport of bilirubin, hormones, minerals (calcium, magnesium, zinc, mercury), fats, etc. These theoretical premises find application in the clinic in the treatment of hyperbilirubinemia characteristic of the neonatal period. To reduce bilirubinemia, the introduction of a pure albumin preparation was shown to prevent toxic effects on the central nervous system - the development of encephalopathy.
Globulins having a high molecular weight (90 000-150 000), refer to complex proteins, which include various complexes. In α1- and α2-globulins are muco-and glycoproteins, which is reflected in inflammatory diseases. The bulk of the antibodies are related to γ-globulins. A more detailed study of γ-globulins showed that they consist of different fractions, the change of which is characteristic of a number of diseases, that is, they also have diagnostic significance.
The study of the protein content and its so-called spectrum, or protein formula of blood, has found wide application in the clinic.
In the body of a healthy person, albumins predominate (about 60% protein). The ratio of globulin fractions is easy to remember: α1-1, α2 -2, β-3, y-4 parts. In acute inflammatory diseases, changes in the protein formula of blood are characterized by an increase in the content of α-globulins, especially due to α2, with a normal or slightly increased content of γ-globulins and a reduced amount of albumins. With chronic inflammation there is an increase in the content of y-globulin at a normal or slightly increased content of α-globulin, a decrease in albumin concentration. Subacute inflammation is characterized by a simultaneous increase in the concentration of α- and γ-globulins with a decrease in the albumin content.
The appearance of hypergammaglobulinemia indicates a chronic period of the disease, hyperalphaglobulinemia - on an exacerbation. In the human body, proteins are digested with hydrolytically peptidases to amino acids, which, depending on the need, are used to synthesize new proteins or are converted to keto acids and ammonia by deamination. In children in the blood serum, the amino acid content approaches the values characteristic of adults. Only in the first days of life there is an increase in the content of certain amino acids, which depends on the type of feeding and the relatively low activity of the enzymes involved in their metabolism. In this regard, aminoaciduria in children is higher than in adults.
In newborns, physiological azotemia (up to 70 mmol / l) is observed in the first days of life. After the maximum increase to the 2nd-3rd day of life, the level of nitrogen decreases and reaches the level of an adult person (28 mmol / l) by the 5-12th day of life. In preterm infants, the level of residual nitrogen is higher the lower the weight of the child. Azotemia during this period of childhood is associated with excision and insufficient kidney function.
The protein content of food significantly affects the level of residual blood nitrogen. Thus, when the protein content in the food is 0.5 g / kg, the urea concentration is 3.2 mmol / l, at 1.5 g / kg 6.4 mmol / l, at 2.5 g / kg - 7.6 mmol / l . To some extent, an indicator reflecting the state of protein metabolism in the body is the excretion of the final products of protein metabolism in the urine. One of the important end products of protein metabolism - ammonia - is a toxic substance. It is rendered harmless:
- by isolating ammonium salts through the kidneys;
- transformation into non-toxic urea;
- by binding to α-ketoglutaric acid in glutamate;
- binding with glutamate under the action of the enzyme glutamine synthetase in glutamine.
In an adult human products of nitrogen metabolism are excreted in the urine, mainly in the form of a low-toxic urea, the synthesis of which is carried out by the cells of the liver. Urea in adults is 80% of the total amount of nitrogen excreted. In newborns and children of the first months of life, the percentage of urea is lower (20-30% of total urine nitrogen). In children under 3 months of urea, 0.14 g / (kg-day) is released, 9-12 months-0.25 g / (kg-day). In a newborn a significant amount in the total urine nitrogen is uric acid. Children up to 3 months of life allocate 28.3 mg / (kg-day), and adults - 8.7 mg / (kg-day) of this acid. Excessive its content in the urine is the cause of uric acid infarcts of the kidneys, which are observed in 75% of newborns. In addition, the organism of the child of early age displays nitrogen of the protein in the form of ammonia, which in urine is 10-15%, and in an adult - 2.5-4.5% of total nitrogen. This is explained by the fact that in children of the first 3 months of life the liver function is not developed enough, so excessive protein load can lead to the appearance of toxic exchange products and their accumulation in the blood.
Creatinine excreted in the urine. Isolation depends on the development of the muscular system. In premature infants, 3 mg / kg of creatinine are released per day, 10-13 mg / kg in full-term newborns, and 1.5 g / kg in adults.
Disturbance of protein metabolism
Among the various congenital diseases, which are based on the violation of protein metabolism, a significant proportion have amino acid fractures, which are based on a deficiency of enzymes involved in their metabolism. Currently, more than 30 different forms of aminoacidopathy are described. Their clinical manifestations are very diverse.
Relatively frequent manifestations of aminoacidopathies are neuropsychiatric disorders. The lag of the neuropsychic development in the form of various degrees of oligophrenia is characteristic of many aminoacidopathies (phenylketonuria, homocystinuria, histidemia, hyperammonemia, citrullineemia, hyperprolinaemia, Hartnup disease, etc.), which is confirmed by their high prevalence exceeding in tens and hundreds of times that in the general population.
Convulsive syndrome is often found in children with aminocidopathies, and convulsions often appear in the first weeks of life. There are often flexor spasms. Especially they are peculiar to phenylketonuria, and also occur in violation of the exchange of tryptophan and vitamin B6 (pyridoxine), with glycinosis, leucinosis, prolinuria, etc.
Often there is a change in muscle tone in the form of hypotension (hyperlysinemia, cystinuria, glycinosis, etc.) or, conversely, hypertension (leucinosis, hyperuricemia, Hartnup disease, homocystinuria, etc.). Change in muscle tone may periodically increase or decrease.
The delay in the development of speech is characteristic of histidemia. Visual disturbances are often found in aminoacidopathies of aromatic and sulfur-containing amino acids (albinism, phenylketonuria, histidinemia), pigment deposition - in alkaponuria, lens dislocation - in homocystinuria.
Changes in the skin with aminoacidopathy are not uncommon. Disturbances (primary and secondary) pigmentation are characteristic of albinism, phenylketonuria, less often histidemia and homocystinuria. Intolerance to insolation (sunburn) in the absence of sunburn is observed with phenylketonuria. Pellagroide skin is characteristic of Hartnup disease, eczema - phenylketonuria. With arginine succinate aminoaciduria, brittle hair is observed.
Gastrointestinal symptoms are very common with aminoacidemia. Difficulty in nutrition, often vomiting, almost from birth, characterized by glycinosis, phenylketonuria, tyrosinose, citrullineemia, etc. Vomiting can be fits and cause rapid dehydration and a co-morbid state, sometimes to someone with convulsions. With a high protein content, there is an increase and more frequent vomiting. With glycinosis, it is accompanied by ketonomy and ketonuria, a violation of breathing.
Often, with arginine succinate aminoaciduria, homocystinuria, hypermethioninemia, tyrosinosis, liver damage is observed, up to the development of cirrhosis with portal hypertension and gastrointestinal bleeding.
With hyperprolinaemia, renal symptoms (hematuria, proteinuria) are noted. There may be changes in blood. Anemias are characterized by hyperlysinemia, and leukopenia and thrombocytopathy are glycinose. With homocystinuria, platelet aggregation may increase with the development of thromboembolism.
Aminoacidemia can occur during the period of newborns (leucinosis, glycinosis, hyperammonia), but the severity of the condition usually increases to 3-6 months because of significant accumulation in patients both amino acids and products of disturbed metabolism. Therefore, this group of diseases can be rightly attributed to accumulation diseases, which causes irreversible changes, primarily the central nervous system, liver and other systems.
Along with the violation of the exchange of amino acids can be observed diseases, which are based on a violation of protein synthesis. It is known that in the nucleus of each cell the genetic information is in chromosomes, where it is encoded in DNA molecules. This information is transferred to the transport RNA (tRNA), which passes into the cytoplasm, where it is translated into the linear sequence of amino acids that make up the polypeptide chains, and protein synthesis occurs. Mutations of DNA or RNA disrupt the synthesis of a protein of the correct structure. Depending on the activity of a specific enzyme, the following processes are possible:
- Lack of formation of the final product. If this connection is vital, then a fatal outcome will follow. If the end product is a compound that is less important for life, these conditions manifest immediately after birth, and sometimes even later. An example of such a disorder is hemophilia (lack of synthesis of antihemophilic globulin or its low content) and afibrinogenemia (low content or absence of fibrinogen in the blood), which are manifested by increased bleeding.
- Accumulation of intermediate metabolites. If they are toxic, then clinical signs develop, for example, in phenylketonuria and other aminoacidopathies.
- Minor metabolic pathways can become major and overloaded, and metabolites formed normally can accumulate and excrete in unusually large quantities, for example, in alkaponuria. To such diseases it is possible to carry hemoglobinopathies, at which the structure of polypeptide chains is changed. More than 300 anomalous hemoglobins have already been described. So, it is known that the adult type of hemoglobin consists of 4 polypeptide chains of aarr, into which amino acids are in a certain sequence (141 chains in the α chain and 146 amino acids in the β chain). It is encoded in the 11th and 16th chromosome. The replacement of glutamine with valine forms hemoglobin S, which has α2-polypeptide chains, in gemoglobin C (α2β2) glycine is replaced with lysine. The whole hemoglobinopathy group is clinically manifested by spontaneous or some kind of hemolytic factor, a changing affinity for oxygen transfer by the heme, often an increase in the spleen.
Insufficiency of the vascular or platelet factor of von Willebrand causes increased bleeding, which is especially common among the Swedish population of the Åland Islands.
To this group should include and various types of macroglobulinemia, as well as a violation of the synthesis of individual immunoglobulins.
Thus, the violation of protein metabolism can be observed at the level of both its hydrolysis and absorption in the gastrointestinal tract, and intermediary metabolism. It is important to emphasize that violations of protein metabolism, as a rule, are accompanied by violation of other types of metabolism, since the composition of almost all enzymes includes the protein part.