Medical expert of the article
New publications
Lasers in plastic surgery
Last reviewed: 23.04.2024
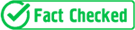
All iLive content is medically reviewed or fact checked to ensure as much factual accuracy as possible.
We have strict sourcing guidelines and only link to reputable media sites, academic research institutions and, whenever possible, medically peer reviewed studies. Note that the numbers in parentheses ([1], [2], etc.) are clickable links to these studies.
If you feel that any of our content is inaccurate, out-of-date, or otherwise questionable, please select it and press Ctrl + Enter.
At the beginning of the last century, in a publication titled "Quantum Theory of Radiation," Einstein theoretically substantiated the processes that must take place when the laser emits energy. Maiman built the first laser in 1960. Since then, the rapid development of laser technology, leading to the creation of a variety of lasers, covering the entire electromagnetic spectrum. Then they merged with other technologies, including visualization systems, robotics and computers, to improve the accuracy of the transmission of laser radiation. As a result of cooperation in the field of physics and bioengineering, medical lasers as therapeutic agents have become an important part of the arsenal of surgeons. Initially, they were cumbersome and were used only by surgeons who were specially trained in the physics of lasers. Over the past 15 years, the design of medical lasers has advanced in the direction of ease of use, and many surgeons have studied the basics of laser physics in postgraduate education.
This article discusses: the biophysics of lasers; interaction of tissues with laser radiation; devices currently used in plastic and reconstructive surgery; general safety requirements for working with lasers; questions of the further application of lasers at interventions on the skin.
Biophysics of lasers
Lasers emit light energy, which moves in the form of waves similar to ordinary light. The wavelength is the distance between two adjacent wave highs. Amplitude is the magnitude of the maximum, determines the intensity of the light radiation. The frequency, or the period of the light wave, is the time required for one complete wave cycle. To understand the effect of a laser, it is important to consider quantum mechanics. The term "laser" (LASER) is an abbreviation of the phrase "light amplification by Stimulated Emission of Radiation". If a photon, a unit of light energy, collides with an atom, it transfers one of the electrons of the atom to a higher energy level. The atom in such an excited state becomes unstable and again releases a photon when the electron passes to the initial, lower energy level. This process is known as spontaneous emission. If an atom is in a high-energy state and collides with another photon, then, upon transition to a low-energy level, it will allocate two photons that have the same wavelength, direction and phase. This process, called stimulated emission of radiation, underlies the understanding of laser physics.
Regardless of the type, all lasers have four main components: an exciting mechanism or an energy source, a laser medium, an optical cavity or a resonator, and an ejection system. Most medical lasers used in facial plastic surgery have an electrical excitation mechanism. Some lasers (for example, a dye laser excited by a flash lamp) use light as the excitation mechanism. Others can use high-energy radio waves or chemical reactions to provide excitation energy. The exciter mechanism pumps energy into a resonant chamber containing a laser medium, which may be a solid, liquid, gaseous or semi-conductive material. The energy discharged into the cavity of the resonator raises the electrons of the atoms of the laser medium to a higher energy level. When half the atoms in the resonator reach high excitation, the population inversion occurs. Spontaneous emission begins when photons are emitted in all directions and some of them collide with already excited atoms, which leads to stimulated emission of pair photons. The amplification of the stimulated emission occurs as the photons moving along the axis between the mirrors are reflected mainly back and forth. This leads to successive stimulation, since these photons collide with other excited atoms. One mirror has 100% reflection, and the other - partially transmits the radiated energy from the cavity chamber. This energy is transferred to the biological tissues by the ejection system. In most lasers it is fiber-optic. A notable exception is the C02 laser, which has a system of mirrors on a hinged bar. For the C02 laser there are optical fibers, but they limit the spot size and the output energy.
The light of the laser in comparison with ordinary light is more organized and qualitatively intensive. Since the laser medium is homogeneous, the photons emitted under stimulated emission have one wavelength, which creates monochromaticity. Usually, light diffuses strongly as it moves away from the source. Laser light is collimated: it dissipates little, providing a constant intensity of energy at a great distance. Photons of laser light not only move in one direction, they have the same temporal and spatial phase. This is called coherence. The properties of monochromaticity, collimation and coherence distinguish laser light from the disordered energy of ordinary light.
Laser-tissue interaction
The spectrum of laser effects on biological tissues extends from the modulation of biological functions to evaporation. Most clinically used laser-tissue interactions involve thermal coagulation or evaporation. In the future, lasers can be used not as heat sources, but as probes for controlling cellular functions without side effects of cytotoxic effects.
The effect of an ordinary laser on tissue depends on three factors: tissue absorption, laser wavelength, and laser energy density. When a laser beam collides with a tissue, its energy can be absorbed, reflected, transmitted or scattered. With any interaction of tissue and laser, all four processes occur to varying degrees, of which absorption is the most important. The degree of absorption depends on the content of the chromophore in the tissue. Chromophores are substances that effectively absorb waves of a certain length. For example, the energy of the CO2 laser is absorbed by the soft tissues of the body. This is due to the fact that the wavelength corresponding to C02 is well absorbed by water molecules, which constitute up to 80% of soft tissues. In contrast, the C02 laser is minimally absorbed by the bone, which is due to the low water content in the bone tissue. Initially, when the tissue absorbs laser energy, its molecules begin to vibrate. Absorption of additional energy causes denaturation, coagulation and, finally, evaporation of the protein (vaporization).
When the laser energy is reflected by the tissue, the latter is not damaged, since the radiation direction on the surface changes. Also, if the laser energy passes through the surface tissues into the deep layer, the intermediate tissue is not affected. If the laser beam dissipates into the tissue, the energy is not absorbed on the surface, but randomly distributed in the deep layers.
The third factor concerning the interaction of tissues with a laser is the energy density. When the laser and tissue interact, when all other factors are constant, changing the size of the spot or exposure time can affect the tissue state. If the size of the spot of the laser beam decreases, the power acting on a certain volume of tissue increases. Conversely, if the spot size increases, the energy density of the laser beam decreases. To change the size of the spot, you can focus, pre-focus or defocus the ejection system on the fabric. With the prefocusing and defocusing of the rays, the spot size is larger than the focused beam, which results in a lower power density.
Another way to change the tissue effects is the pulsation of laser energy. All pulse modes of radiation intermittent periods of power on and off. Since the energy does not reach the tissue during the shutdown periods, it is possible to dissipate heat. If the shut-off periods are longer than the thermal relaxation time of the target tissue, the probability of damage to the surrounding tissue by thermal conductivity decreases. The thermal relaxation time is the amount of time required to dissipate half the heat of an object. The ratio of the duration of the active gap to the sum of the active and passive pulsation intervals is called the duty cycle.
Operating cycle = on / on + off
There are various pulse modes. Energy can be produced in batches by setting the period when the laser emits (eg, OD c). Energy can overlap when a constant wave is blocked at certain intervals by a mechanical shutter. In the super pulse mode, the energy is not simply blocked, but stored in the laser energy source during the shutdown period, and then ejected during the on-period. That is, the peak energy in the super-pulse mode is significantly higher than that in constant mode or overlap mode.
In a laser generating in the giant pulse regime, the energy is also conserved during the shutdown period, but in a laser environment. This is achieved by using a damper mechanism in the cavity chamber between the two mirrors. A closed flap prevents generation in the laser, but allows energy to be stored on each side of the flap. When the flap is open, the mirrors interact, causing the formation of a high-energy laser beam. The peak energy of a laser generating in the giant pulse regime is very high with a short operating cycle. A laser with synchronized modes is similar to a laser that generates in the giant pulse mode, in that a damper is provided between the two mirrors in the cavity chamber. A laser with synchronized modes opens and closes its damper in synchronization with the time it takes to reflect light between two mirrors.
Characteristics of lasers
- Carbon dioxide laser
Carbon dioxide laser is most often used in otorhinolaryngology / head and neck surgery. The length of its wave is 10.6 nm - an invisible wave of the far infrared region of the spectrum of electromagnetic radiation. Guidance along the beam of a helium-neon laser is necessary in order for the surgeon to see the area of influence. The laser medium is C02. Its wavelength is well absorbed by water molecules in the tissue. The effects are superficial due to high absorption and minimal dispersion. Radiation can only be transmitted through mirrors and special lenses placed on a hinged bar. The crank bar can be attached to the microscope for precision work under magnification. Energy can also be ejected through a focusing handle attached to the hinge bar.
- Nd: YAG laser
The wavelength of the Nd: YAG (yttrium-aluminum garnet with neodymium) laser is 1064 nm, that is, it is in the near infrared region. It is invisible to the human eye and requires a suggestive helium-neon laser beam. The laser medium is yttrium-aluminum garnet with neodymium. Most body tissues do not absorb this wavelength well. However, the pigmented tissue absorbs it better than the unpigmented one. Energy is transmitted through the surface layers of most tissues and is dispersed in deep layers.
Compared with a carbon dioxide laser, the scattering of Nd: YAG is much larger. Therefore, the depth of penetration is greater and Nd: YAG is well suited for coagulation of deeply lying vessels. In the experiment, the maximum depth of coagulation is about 3 mm (coagulation temperature +60 ° C). Good results of treatment of deep perioral capillary and cavernous formations with the help of Nd: YAG laser have been reported. There is also a report on successful laser photocoagulation with hemangiomas, lymphangiomas and arteriovenous congenital formations. However, a greater depth of penetration and indiscriminate destruction predispose to an increase in postoperative scarring. Clinically, this is minimized by safe power settings, a point approach to the outbreak and avoidance of skin areas. In practice, the use of a dark red Nd: YAG laser was practically replaced by lasers with a wavelength lying in the yellow part of the spectrum. However, it is used as an auxiliary laser for nodal formations of dark red color (port color).
It has been shown that the Nd: YAG laser suppresses the production of collagen, both in fibroblast culture and in normal skin in vivo. This suggests the success of this laser in the treatment of hypertrophic scars and keloids. But clinically the frequency of relapse after keloids is high, despite the powerful additional local treatment with steroids.
- Contact Nd: YAG laser
The use of the Nd: YAG laser in the contact mode significantly changes the physical properties and absorptivity of the radiation. The contact tip consists of a crystal of sapphire or quartz, directly attached to the end of the laser fiber. The contact tip interacts directly with the skin and acts as a thermal scalpel, cutting and coagulating simultaneously. There are reports of the use of a contact tip with a wide range of interventions on soft tissues. These applications are closer to electrocoagulation than non-contact Nd: YAG. Basically, surgeons now use laser-specific wavelengths not for cutting tissues, but for heating the tip. Therefore, the principles of interaction of the laser with tissues are not applicable here. The response time to the contact laser is not as direct a function as when using a free fiber, and therefore there is a lag period for heating and cooling. However with experience this laser becomes convenient for allocation of skin and muscle grafts.
- Argon laser
The argon laser emits visible waves with a length of 488-514 nm. Due to the design of the cavity chamber and the molecular structure of the laser medium, this type of laser produces a long-wavelength range. Individual models may have a filter that limits radiation to a single wavelength. The energy of the argon laser is well absorbed by hemoglobin, and its dispersion is intermediate between the carbon dioxide and Nd: YAG laser. The radiation system for an argon laser is a fiber optic carrier. Because of the large absorption by hemoglobin, the vascular neoplasms of the skin also absorb the energy of the laser.
- KTP laser
The KTP (potassium titanyl phosphate) laser is an Nd: YAG laser whose frequency is doubled (the wavelength is halved) by passing laser energy through the KT crystal. This gives green light (wavelength 532 nm), which corresponds to the absorption peak of hemoglobin. Its penetration into tissues and scattering is similar to that of an argon laser. Laser energy is transferred by fiber. In non-contact mode, the laser evaporates and coagulates. In the semi-contact mode, the tip of the fiber barely touches the fabric and becomes a cutting tool. The more energy is used, the more the laser acts as a thermal knife, similar to a carbon-acid laser. Installations with lower energy are used primarily for coagulation.
- A dye laser excited by a flash lamp
The dye laser excited by the flash lamp was the first medical laser specially developed to treat benign vascular neoplasms of the skin. This is a visible light laser with a wavelength of 585 nm. This wavelength coincides with the third peak of absorption by oxyhemoglobin, and therefore the energy of this laser is predominantly absorbed by hemoglobin. In the 577-585 nm range, there is also less absorption by competing chromophores, such as melanin, and less scattering of laser energy in the dermis and epidermis. The laser medium is dye rhodamine, which is optically excited by a flash lamp, and the radiation system is a fiber optic carrier. The tip of the dye laser has a replaceable lens system, which allows to create a spot size of 3, 5, 7 or 10 mm. The laser pulsates with a period of 450 ms. This pulsation index was chosen based on the thermal relaxation time of ectatic vessels found in benign vascular neoplasms of the skin.
- Copper vapor laser
A copper vapor laser produces visible radiation having two separate wavelengths: a pulsed green wave of 512 nm in length and a pulsed yellow wave of 578 nm in length. The laser medium is copper, which is excited (evaporated) electrically. The fiber-fiber system transfers energy to the tip, which has a variable spot size of 150-1000 μm. Exposure time ranges from 0.075 s to a constant. The time between pulses also varies from 0.1 s to 0.8 s. Yellow copper vapor laser light is used to treat benign vascular lesions on the face. The green wave can be used to treat such pigmented formations as freckles, lentigo, nevi and keratosis.
- Non-damped yellow dye laser
A yellow dye laser with an undamped wave is a visible light laser producing yellow light with a wavelength of 577 nm. Like a laser on a dye, excited by a flash lamp, it is tuned by changing the dye in the laser activation chamber. The dye is excited by an argon laser. The ejection system for this laser is also fiber optic cable, which can be focused on different spot sizes. The laser light can pulsate by using a mechanical shutter or a Hexascanner tip attached to the end of the fiber optic system. Hexascanner randomly directs pulses of laser energy inside the hexagonal contour. Like a dye laser excited by a flash lamp, and a copper vapor laser, a yellow dye laser with undamped wave is ideally suited for treating benign vascular lesions on the face.
- Erbium laser
Erbium: The UAS laser uses a band of absorption spectrum with water of 3000 nm. Its wavelength of 2940 nm corresponds to this peak and is strongly absorbed by the tissue water (about 12 times larger than the carbon dioxide laser). This laser, emitting in the near-infrared spectrum, is invisible to the eye and should be used with a visible guide beam. The laser is pumped by a flash lamp and emits macro-pulses of 200-300 μs duration, which consist of a series of micropulse. These lasers are used with a tip attached to the hinge bar. A scanning device can also be integrated in the system for faster and more uniform removal of tissue.
- Ruby laser
Ruby laser - a laser pumped by a pulsed lamp emitting light with a wavelength of 694 nm. This laser, located in the red region of the spectrum, is visible with the eye. It can have a laser shutter to produce short pulses and achieve a deeper penetration into the tissue (deeper than 1 mm). A long-pulse ruby laser is used to preferentially heat the hair follicles during laser hair removal. This laser radiation is transmitted by means of mirrors and the system of a hinged rod. It is poorly absorbed by water, but strongly absorbed by melanin. Different pigments used for tattoos also absorb rays with a wavelength of 694 nm.
- Alexandrite laser
The Alexandrite laser, a solid-state laser that can be inflated by a flash lamp, has a wavelength of 755 nm. This wavelength, located in the red part of the spectrum, is not visible to the eye and therefore requires a guiding beam. It is absorbed by blue and black pigments for tattoos, as well as melanin, but not hemoglobin. This is a relatively compact laser that can transmit radiation over a flexible fiber. The laser penetrates relatively deep, which makes it convenient for removing hair and tattoos. The spot size is 7 and 12 mm.
- Diode laser
Recently, diodes on superconducting materials were directly coupled with fiber optic devices, which led to the emission of laser radiation with different wavelengths (depending on the characteristics of the materials used). Diode lasers are distinguished by their performance. They can transfer incoming electrical energy into the light with an efficiency of 50%. This efficiency, associated with less heat generation and input power, allows compact diode lasers to have a design devoid of large cooling systems. The light is transmitted fiber optically.
- Filtered Impulse Lamp
The filtered pulse lamp used for hair removal is not a laser. On the contrary, it is an intense, incoherent, impulse spectrum. For the emission of light with a wavelength of 590-1200 nm, the system uses crystal filters. The width and integral density of the pulse, also variable, satisfy the criteria for selective photothermolysis, which puts this device on a par with hair removal lasers.