Medical expert of the article
New publications
Free radicals and antioxidants
Last reviewed: 23.04.2024
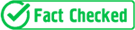
All iLive content is medically reviewed or fact checked to ensure as much factual accuracy as possible.
We have strict sourcing guidelines and only link to reputable media sites, academic research institutions and, whenever possible, medically peer reviewed studies. Note that the numbers in parentheses ([1], [2], etc.) are clickable links to these studies.
If you feel that any of our content is inaccurate, out-of-date, or otherwise questionable, please select it and press Ctrl + Enter.
The discovery of free radicals and antioxidants has become just as important for medical science as the discovery of microorganisms and antibiotics at one time, because doctors have not only explained many pathological processes, including aging, but also effective methods of combating them.
The last decade was marked by successes in the study of free radicals in biological objects. These processes proved to be a necessary metabolic link in the normal vital activity of the body. They participate in the reactions of oxidative phosphorylation, in the biosynthesis of prostaglandins and nucleic acids, in the regulation of lipotic activity, in the processes of cell division. In the body free radicals are most often formed during the oxidation of unsaturated fatty acids, and this process is closely related to peroxidant free radical lipid oxidation (LPO).
What is free radicals?
A free radical is a molecule or atom that has an unpaired electron in an external orbit, which causes its aggressiveness and the ability not only to react with the molecules of the cell membrane, but also to convert them into free radicals (self-sustaining avalanche reaction).
Carbon containing the radical reacts with molecular oxygen, forming a peroxide free radical of the COO.
The peroxide radical extracts hydrogen from the side chain of unsaturated fatty acids, forming a lipid hydroperoxide and another carbon containing a radical.
Lipid hydroperoxides increase the concentration of cytotoxic aldehydes, and the carbon containing the radical supports the formation of peroxide radicals, etc. (along the chain).
Various mechanisms of the formation of free radicals are known. One of them is the effect of ionizing radiation. In some situations, in the process of molecular oxygen reduction, one electron is added instead of two, and a highly reactive superoxide anion (O) is formed. The formation of superoxide is one of the protective mechanisms against bacterial infection: without oxygen free radicals, neutrophils and macrophages can not kill bacteria.
The presence of antioxidants, both in the cell and in the extracellular space, indicates that the formation of free radicals is not an episodic phenomenon caused by exposure to ionizing radiation or toxins, but a constant accompanying oxidation reactions under normal conditions. The main antioxidants are the enzymes of the superoxide dismutase group (SODs), whose function is to catalyze the peroxide anion into hydrogen peroxide and molecular oxygen. Since superoxide dismutases are ubiquitous, it is legitimate to assume that the superoxide anion is one of the main by-products of all oxidation processes. Catalases and peroxidases convert the hydrogen peroxide formed during the process of dismutation into water.
The main feature of free radicals is their unusual chemical activity. As if feeling their defectiveness, they try to regain the lost electron, aggressively taking it away from other molecules. In turn, "offended" molecules also become radicals and already begin to rob themselves, taking away electrons from their neighbors. Any changes in the molecule-be it the loss or addition of an electron, the appearance of new atoms or groups of atoms-affect its properties. Therefore, free-radical reactions taking place in a substance, change the physicochemical properties of this substance.
The best-known example of a free radical process is oil spoilage (rancidity). Rancid oil has a peculiar taste and smell, which is explained by the appearance in it of new substances formed during free radical reactions. Most importantly, the participants of free radical reactions can become proteins, fats and DNA of living tissues. This leads to the development of a variety of pathological processes that damage tissues, aging and development of malignant tumors.
The most aggressive of all free radicals are free radicals of oxygen. They can provoke an avalanche of free radical reactions in living tissue, the consequences of which can be catastrophic. Free radicals of oxygen and its active forms (for example, lipid peroxides) can form in the skin and any other tissue under the influence of UV radiation, some toxic substances contained by water and air. But the most important thing is that active forms of oxygen are formed with any inflammation, any infectious process occurring in the skin or any other organ, since they are the main weapon of the immune system, by which it destroys pathogenic microorganisms.
To hide from free radicals it is impossible (also as it is impossible to disappear from bacteria but from them it is possible to be protected). There are substances that differ in that their free radicals are less aggressive than radicals of other substances. Having given its electron to the aggressor, the antioxidant does not seek to compensate for the loss due to other molecules, or rather, it does this only in rare cases. Therefore, when a free radical reacts with an antioxidant, it turns into a full-fledged molecule, and the antioxidant becomes a weak and inactive radical. Such radicals are already harmless and do not create chemical chaos.
What are antioxidants?
"Antioxidants" is a collective concept and, like concepts such as "antiloblastics" and "immunomodulators", does not imply membership of any particular chemical group of substances. Their specificity is the closest relationship with free radical lipid oxidation in general and free radical pathology in particular. This property combines various antioxidants, each of which has its own peculiarities of action.
The processes of free radical lipid oxidation are of a general biological nature and, when they are sharply activated, according to many authors, a universal mechanism of cell damage at the membrane level. In the lipid phase of biological membranes lipoperoxidation processes cause an increase in the viscosity and order of the membrane bilayer, change the phase properties of membranes and reduce their electrical resistance, and facilitate the exchange of phospholipids between two monolayers (the so-called phospholipid flip-flop). Under the influence of peroxide processes, there is also an inhibition of the mobility of membrane proteins. At the cellular level, lipid peroxidation is accompanied by swelling of mitochondria, dissociation of oxidative phosphorylation (and in the case of a far-gone process, solubilization of membrane structures), which at the level of the whole organism is manifested in the development of so-called free radical pathologies.
Free radicals and cell damage
Today it became evident that the formation of free radicals is one of the universal pathogenetic mechanisms for various types of cell damage, including the following:
- reperfusion of cells after a period of ischemia;
- some medicamentally induced forms of hemolytic anemia;
- poisoning with some herbicides;
- the management of carbon tetrachloride;
- ionizing radiation;
- some mechanisms of cell aging (for example, the accumulation of lipid products in the cell - of ceroids and lipofuscin);
- Oxygen toxicity;
- atherogenesis due to oxidation of low density lipoproteins in arterial wall cells.
Free radicals participate in the processes:
- aging;
- carcinogenesis;
- chemical and drug damage of cells;
- inflammation;
- radioactive damage;
- atherogenesis;
- oxygen and ozone toxicity.
Effects of free radicals
Oxidation of unsaturated fatty acids in the composition of cell membranes is one of the main effects of free radicals. Free radicals also damage proteins (especially thiol-containing proteins) and DNA. The morphological outcome of lipid oxidation of the cell wall is the formation of polar permeability channels, which increases the passive permeability of the membrane for Ca2 + ions, the excess of which is deposited in the mitochondria. Oxidation reactions are usually suppressed by hydrophobic antioxidants, such as vitamin E and glutathione peroxidase. Vitamin E-like antioxidants that break oxidation chains are found in fresh vegetables and fruits.
Free radicals also react with molecules in the ionic and aqueous environments of cellular compartments. In the ionic medium, the antioxidant potential is retained by molecules of substances such as reduced glutathione, ascorbic acid, and cysteine. The protective properties of antioxidants become evident when, when depleted of their stocks in an isolated cell, characteristic morphological and functional changes are observed, due to the oxidation of lipids of the cell membrane.
The types of damage caused by free radicals are determined not only by the aggressiveness of the produced radicals, but also by the structural and biochemical characteristics of the target. For example, in the extracellular space, free radicals destroy glycosaminoglycans of the main substance of connective tissue, which can be one of the mechanisms of joint destruction (for example, in rheumatoid arthritis). Free radicals change the permeability (and hence the barrier function) of cytoplasmic membranes in connection with the formation of channels of increased permeability, which leads to disruption of the water-ion homeostasis of the cell. It is believed that the availability of vitamins and microelements in patients with rheumatoid arthritis is necessary, in particular, the correction of vitamin deficiency and micronutrient deficiency by oligogal E. This is due to the fact that the significant activation of the processes of peroxidation and inhibition of antioxidant activity is proved. Therefore, it is very important to include in the complex therapy bioantioxidants with high antiradical activity, to which vitamins antioxidants (E, C and A) and trace elements of selenium (Se) belong. It is also shown that the use of a synthetic dose of vitamin E, which is absorbed worse than natural. For example, doses of vitamin E to 800 and 400 IU / day lead to a decrease in cardiovascular diseases (by 53%). However, the response to the effectiveness of antioxidants will be obtained in large controlled trials (from 8,000 to 40,000 patients), which were conducted in 1997.
As protective forces that maintain the LPO rate at a certain level, enzyme systems for inhibiting oxidation peroxidation and natural antioxidants are isolated. There are 3 levels of regulation of the rate of free radical oxidation. The first stage is anoxic, maintaining a fairly low oxygen partial pressure in the cell. This includes primarily respiratory enzymes that compete for oxygen. Despite the wide variability in the absorption of O3 in the body and the release of CO2, pO2 and pCO2 in the arterial blood from it, the norm is fairly constant. The second stage of defense is anti-radical. It consists of various substances in the body (vitamin E, ascorbic acid, certain steroid hormones, etc.), which interrupt the processes of LPO, interacting with free radicals. The third stage is antiperoxide, which destroys the peroxides already formed with the help of appropriate enzymes or non-enzymatically. However, there is still no single classification and unified views on the mechanisms of regulation of the speed of free radical reactions and the action of protective forces that ensure the disposal of the final products of LPO.
It is believed that depending on the intensity and duration, the changes in the regulation of the reactions of LPO can: first, be reversible with subsequent return to normal, second, lead to a transition to another level of autoregulation and, thirdly, some of the impacts this mechanism of self-regulation is disunited, and, consequently, lead to the impossibility of exercising regulatory functions. That is why the understanding of the regulatory role of LPO reactions in conditions of extreme factors, in particular, the cold, is an essential stage of research aimed at developing scientifically based methods of managing adaptation processes and complex therapy, prevention and rehabilitation of the most common diseases.
One of the most commonly used and effective is a complex of antioxidants, which includes tocopherol, ascorbate and methionine. Analyzing the mechanism of action of each of the antioxidants used, the following is noted. Microsomes - one of the main places of accumulation in the cells of the liver of exogenously introduced tocopherol. As a possible donor of protons, ascorbic acid can act, which is oxidized to dehydroascorbic acid. In addition, the ability of ascorbic acid to interact directly with singlet oxygen, a hydroxyl radical and a superoxide radical anion, and also destroy hydrogen peroxide is shown. There is also evidence that tocopherol in microsomes can be regenerated by thiols and, in particular, reduced glutathione.
Thus, the body has a number of interrelated antioxidant systems, the main role of which is to maintain enzymatic and non-enzymatic oxidative reactions at a stationary level. At each stage of development of peroxide reactions there is a specialized system that implements these functions. Some of these systems are strictly specific, others, such as glutathione peroxidase, tocopherol, have a greater range of action and less substrate specificity. The additivity of the interaction of enzymatic and non-enzymatic antioxidant systems with each other ensures the stability of the organism to extreme factors that have prooxidant properties, ie, the ability to create conditions in the body predisposing to the development of activated oxygen forms and the activation of LPO reactions. There is no doubt that the activation of LPO reactions is observed under the influence of a number of environmental factors on the body and pathological processes of a different nature. According to V. Yu. Kulikov et al. (1988), depending on the mechanisms of activation of LPO reactions, all the factors acting on the body can be divided into the following groups with a certain probability.
Factors of the physico-chemical nature that promote the increase in the tissues of precursors and direct activators of LPO reactions:
- oxygen under pressure;
- ozone;
- Nitric oxide;
- ionizing radiation, etc.
Factors of biological nature:
- processes of phagocytosis;
- destruction of cells and cell membranes;
- generation system of activated oxygen species.
Factors determining the activity of the antioxidant systems of the organism are enzymatic and non-enzymatic in nature:
- activity of processes associated with the induction of antioxidant systems of enzymatic nature;
- genetic factors associated with the depression of a particular enzyme that regulates the reactions of LPO (deficiency of glutathione peroxidase, catalase, etc.);
- food factors (lack of food in tocopherol, selenium, other trace elements, etc.);
- structure of cell membranes;
- the nature of the relationship between antioxidants of enzymatic and non-enzymatic nature.
Risk factors that potentiate the activation of LPO reactions:
- activation of the oxygen regime of the body;
- the state of stress (cold, high fever, hypoxia, emotional and painful effects);
- hyperlipidemia.
Thus, the activation of LPO reactions in the body is closely related to the functioning of the transport and oxygen utilization systems. Special attention should be paid to adaptogens, among them widely used Eleutherococcus. The drug from the root of this plant has a general strengthening, adaptogenic, anti-stress, antiatherosclerotic, antidiabetic and other properties, reduces the overall incidence, including influenza. When studying the biochemical mechanisms of the action of antioxidants in humans, animals and plant organisms, the spectrum of pathological conditions significantly increased, for the treatment of which antioxidants are used. Antioxidants are successfully used as adaptogens for protection from radiation damage, treatment of wounds and burns, tuberculosis, cardiovascular diseases, neuropsychiatric disorders, neoplasms, diabetes, etc. Naturally, interest has increased in the mechanisms underlying this versatility of antioxidants.
It has now been experimentally established that the effectiveness of antioxidants is determined by their activity in inhibiting lipid peroxidation due to interaction with peroxide and other radicals that initiate LPO, as well as due to the influence of antioxidants on the membrane structure facilitating access of oxygen to lipids. LPO can also change with the mediated system of antioxidant action through neurohormonal mechanisms. It is shown that antioxidants affect the release of the neurotransmitter and the release of hormones, the sensitivity of receptors and their binding. In turn, the change in the concentration of hormones and neurotransmitters changes the intensity of LPO in target cells, which leads to a change in the rate of catabolism of lipids and, as a consequence, to a change in their composition. The relationship between the rate of LPO and the change in the phospholipid spectrum of membranes plays a regulatory role. A similar system of regulation is found in the cell membranes of animals, plant and microbial organisms. As is known, the composition and fluidity of membrane lipids affect the activity of membrane proteins, enzymes, and receptors. Through this system of regulation, antioxidants act on membrane repair, altered in the pathological state of the organism, normalize its composition, structure and functional activity. The change in the activity of enzymes in the synthesis of macromolecules and the composition of the nuclear matrix with a change in the composition of lipids of membranes, caused by the action of antioxidants, can be explained by their effect on the synthesis of DNA, RNA, and protein. At the same time, there appeared in the literature data on the direct interaction of antioxidants with macromolecules.
These, as well as recent data on the effectiveness of antioxidants in picomolar concentrations, highlight the role of receptor pathways in their action on cellular metabolism. In VE Kagan (1981) on the mechanisms of structural and functional modification of biomembranes, it was shown that the dependence of the rate of LPO reactions in biomembranes depends not only on their fatty acid composition (the degree of unsaturation), but also on the structural organization of the lipid phase of membranes (molecular mobility of lipids , strength of protein-lipid and lipid-lipid interactions). It was found that as a result of accumulation of lipid peroxidation products lipid redistribution occurs in the membrane: the amount of liquid lipsts in the biosample decreases, the amount of lipids immobilized by membrane proteins decreases and the amount of ordered lipids in the biosample (clusters) increases. AT.
When studying the nature, composition and mechanism of the homeostasis of the antioxidant system, it is shown that the manifestation of the damaging effect of free radicals and peroxide compounds is hampered by a complex multicomponent antioxidant system (AOC), which provides binding and modification of radicals, prevention of formation or destruction of peroxides. It includes: hydrophilic and hydrophobic organic substances with reducing properties; Enzymes that support the homeostasis of these substances; antiperoxide enzymes. Among the natural antioxidants are lipid (steroid hormones, vitamins E, A, K, flavonoids and polyphenols vitamin P, ubiquinone) and water-soluble (low molecular weight thiols, ascorbic acid) substances. These substances are either traps of free radicals, or destroy peroxide compounds.
One part of the tissue antioxidants is hydrophilic, the other is hydrophobic in nature, which makes it possible to simultaneously protect against oxidizing agents of functionally important molecules in both the aqueous and lipid phases.
The total amount of bioantioxidants creates in the tissues a "buffer antioxidant system" with a certain capacity, and the ratio of prooxidant and antioxidant systems determines the so-called "antioxidant status" of the organism. There is every reason to believe that among the tissue antioxidants a special place is occupied by thiols. The following facts confirm the evidence: high reactivity of sulfhydryl groups, due to which some thiols are oxidized at a very high rate, the dependence of the rate of oxidative modification of SH groups on their radical surroundings in the molecule. This circumstance makes it possible to isolate from a set of thiol compounds a special group of easily oxidizable substances that fulfill the specific functions of antioxidants: the reversibility of the oxidation of sulfhydryl groups to disulphide groups, which in principle makes it possible to energetically maintain the homeostasis of thiol antioxidants in the cell without activating their biosynthesis; The ability of thiols to exhibit both anti-radical and anti-peroxide effects. The hydrophilic properties of thiols are responsible for their high content in the aqueous phase of the cell and the possibility of protection against oxidative damage of biologically important molecules of enzymes, nucleic acids, hemoglobin, etc. At the same time, the presence of non-polar groups in thiol compounds provides the possibility of manifesting their antioxidant activity in the cell's lipid phase. Thus, along with substances of lipid nature, thiol compounds take a wide part in protecting cellular structures from the effects of oxidizing factors.
Oxidation in the tissues of the body is also affected by ascorbic acid. It, like thiols, is part of AOC, participating in the binding of free radicals and the destruction of peroxides. Ascorbic acid, the molecule of which contains both polar and non-polar moieties, exhibits close functional interaction with SH-glutathione and lipid antioxidants, enhancing the effect of the latter and preventing LPO. Apparently, thiol antioxidants play a dominant role in protecting the basic structural components of biological membranes, such as phospholipids or immersed in the lipid layer of proteins.
In turn, water-soluble antioxidants - thiol compounds and ascorbic acid - show their protective action mainly in the aquatic environment - the cytoplasm of the cell or blood plasma. It should be borne in mind that the blood system is an internal environment that plays a decisive role in nonspecific and specific defense reactions, affecting its resistance and reactivity.
Free radicals in pathology
Until now, the literature discusses the issue of cause-effect relationships in changes in the intensity of lipoperoxidation in the dynamics of the development of diseases. In the opinion of some authors, it is the disturbance of stationarity of this process that is the main cause of these diseases, while others believe that a change in the intensity of lipid peroxidation is a consequence of these pathological processes initiated by completely different mechanisms.
Studies conducted in recent years have shown that changes in the intensity of free radical oxidation accompany diseases of various genesis, which confirms the thesis of the general biological nature of free radical damage to cells. Accumulated enough evidence of the pathogenetic involvement of free radical damage to molecules, cells, organs and the body as a whole and successful treatment with their pharmacological preparations that have antioxidant properties.